Tim Palmer (Oxford): Status and Future of Climate Modeling — #16
Steve Hsu: Hi, this is Steve. My guest in today's podcast is Professor Tim Palmer. Tim is Royal society research professor in climate physics, co-director of the Oxford Martin program on modeling and predicting climate. He was trained in general relativity theory at the University of Oxford but changed fields into climate modeling and meteorology.
He was elected a fellow of the Royal Society, commander of the order of the British empire. He won the World Meteorological Organization Norbert Gerbier-Mumm International Award in 2006, the American Meteorological Society Carl-Gustaf Rossby Research Medal in 2010, and the Institute of Physics Dirac Gold Medal in 2014. He was also elected to the National Academy of Sciences of the United States of America in 2020.
I say all these things, just to give you the bonafides of Tim in his field of modeling and predicting climate. I went to Tim for this interview because I wanted a no-nonsense evaluation of the current status of climate models. What are the open questions? What are the things that we're least confident about? And I think we explored those issues quite thoroughly in our discussion.
This was taped in 2020. However, the issues, the state of the field really has not changed much, in the intervening two years. And so, I consider it still an accurate description of the state of climate modeling. I hope you enjoy the interview.
--
Steve Hsu: So, let me start, I always like to get some personal details before we get into science. How did you, what was your path like from being a relativist to, I think, into weather prediction, you worked on weather modeling, and then finally into climate? Maybe you could just describe that for us.
Tim Palmer: Well, yeah, I mean, it's a long story.
Let me start by saying, you know, Einstein and general relativity were kind of a childhood ambition of mine to do some research in that area. I kind of learned about his stuff. I don't know how old I was. 12, 13, 14, and I just became kind of utterly entranced by it. So, I'd set my sight at a, at an early age to try to do a Ph.D. in relativity theory, which I succeeded in doing and then was faced with the, you know, difficult problem, you know, is this something I want to spend my whole life doing?
And I guess I kind of decided, I'd kind of got my childhood ambition out, out, you know, out of my system because I had the Ph.D. I wasn't completely sure whether this was because it was so kind of abstruse and very difficult to communicate. I would say to the person in the street. They'd all say, oh, gee whiz, you know, you're doing black holes. That's fantastic. You know, tell me a bit more about it. And if I did, that would shut them up pretty quickly.
I learned about the weather. I didn't know anything about weather, but, by a strange coincidence, I'd been working on a rather sort of — you know, this is at the time when Steven Hawking announced the very famous result, now that black holes evaporate, they, they lose mass by quantum processes — that people were trying to understand, you know, how to understand this result.
It kind of came out of a very complicated calculation he did. Anyway, I started working on a thing called the principle of maximum entropy production as a way to try to understand Hawking's evaporation. And I met by chance a meteorologist, quite a sort of eminent meteorologist. And I kind of asked him casually, well, what's interesting in your field. And he said, completely unprompted. He says, well, people are trying to apply the principle of maximum entropy production to understand how Earth's climate works. So, this suddenly made it an enormous resonance. And so, I thought, you know, hell, this is a, you know, two topics, which you could hardly think are more unlike each other, Hawking's evaporation of black holes and climate, which seemed to be linked by this common kind of mathematical framework.
So, I decided to have a shot at it. So, I said, well, if I can get a job in this, maybe I can try it for a couple of years. And if I don't like it, I'll go back to theoretical physics. So, I, I, I got a job at the UK's Met office, the weather center where this guy who told me about the maximum entropy applying to climate. He worked and, yeah, I found out I enjoyed it, and I worked there for many years. I worked, I spent a little bit of time at the University of Washington in Seattle, in their atmospheric sciences department. And I spent a long time at the place called the European Center for Medium-Range Weather Forecasts.
And I guess one of the main things I've done over the years is to kind of change the thinking in weather and indeed climate prediction from a kind of deterministic concept to a much more probabilistic one, and thinking about uncertainty as a, as a kind of primary variable in prediction.
The transition, you know, actually all transitions I've made have gone more smoothly than you might think at first sight, because in a way, I mean, I always say this, you know, now I'm a professor at Oxford, and I'm hiring people. If people come with PhDs in, in mathematics or theoretical physics, even if they're from a different subject, it's actually not so difficult to transition, you know, as long as you've got the enthusiasm and the, in, you know, the kind of motivation to make that transition. If you have a Ph.D. in string theory or, you know, number theory or something like that, you actually have 90-something percent of the technical knowledge needed. And the rest of it's just learning up some of the jargon and some of the sort of detail process.
Steve Hsu: Having jumped around in my own research work, I agree with you, but I often find that the most difficult part is convincing the people in that other subject that your opinion might be taken seriously. Because they can always point and say, well, you're just a physicist. What do you know about genomics? Or what do you know about AI?
Tim Palmer: Yeah, they probably do that as a, they probably feel threatened and they're doing that as a defense reaction. But I find in, I mean, one great thing actually about, what, you know, weather and climate research is that people realize it's the kind of field where you only make progress. And we might talk about this a bit later. You only really make progress by working collaboratively and interactively. You know, whereas in some of the more theoretical physics stuff, you know, there's a lot of, you know, I want to be the best I want to have, I want to come up with a breakthrough theory. So, there's a lot more kind of secrecy and, and privacy, I think. And, perhaps a less willingness to interact.
So certainly in climate physics I think people really do embrace new people, new ideas, and, and new thinking. So that hasn't been, I, it's not a problem I've encountered very much.
Steve Hsu: Before we leave your personal journey, I just wanted to say that it's, it's a common story for things like Einstein's theory of special relativity, and then maybe a little bit later general relativity to be what I call a kind of strange attractor for bright kids. Because the idea is so fundamental, like what, what is the nature of space and time? Or what is the dynamics of space-time? You can't help but wonder about those things as if you're a kid when you're just learning. If you're a bright kid, when you're just starting to learn, about time, for example. And so, it attracts people, you know, and it's not, I think what you said is not uncommon for someone to say, wow, this general relativity thing is so amazing. I just would like, at some point in my life, to understand this. This is a monumental achievement of the human mind and it's actually true. It describes the universe. So, it's not to me an uncommon way that people get sucked into physics.
Corey Washington: Yeah. I actually spent a whole year at, in undergrad, doing tensor analysis, and, and basically because of an attraction to general relativity. I eventually didn't do anything with it, but I totally agree with you. It's not just the idea, but it's just the results are so beautifully counterintuitive initially that I think you can't help but just be fascinated by it because your initial intuition [unclear]. And when you're told that the world doesn't work like that, it's sort of mind-blowing. Yeah. I think even for non-technical people.
Tim Palmer: Yeah. However, I, if I could just make an important point that comes out of all this discussion, and I had this point, I discussed this with some of my colleagues in, in the climate field because they published a, a kind of a commentary or something in, I think it was in Nature a few years ago, some quite, sort of eminent colleagues of mine.
And they were kind of bemoaning the fact that indeed the brightest, you know, theoretical physics students, or, or mathematics students, you know, would go into things like, you know, string theory or, or cosmology or, or number theory, or, you know, you know, maybe gr and stuff like that. And, you know, the question that they raised in this commentary is, well, what can we do?
Mean given climate is such an important problem these days. What can we do to make climate a more appealing field for these people? You know? So, instead of, yeah, in choosing, choosing black holes or the big bang or something, they would, they would do something on the cloud feedback problem, say, in climate change.
And I said, I said to my colleagues that you are thinking about this completely the wrong way. You know, if I, when I was starting my Ph.D., if you had said to me, oh, I don't think you should do this. I think you should go into a much more practical field and start, you know, doing weather forecasting or something. I would've told them to go away. You know, I said, look, I'm not interested. Just go. I mean, don't even think about it, you know? It's a non-starter because I've set my sights, you know, from age 12 to do this, and that's what I'm going to do.
But what happens as I say, when I finished my Ph.D. and then started thinking, and it wasn't, I'm going to say this in case people are thinking, it wasn't for a lack of job opportunity. I was given a postdoc position in Hawking’s own group in Cambridge. So, I had that opportunity, but I turned it down. And I turned it down because I wanted to do something a bit more practical and useful with my life, the rest of my life. And I think, again, this is not an uncommon thought for people who have, you know, gone through the Ph.D., maybe done a, you know, one postdoc or something. And suddenly they think, well, you know, this is okay, but I need to be doing something that's going to have a bit more impact on my fellow human beings. And this is when they get very passionate about applying their skills into sort of say environmental sciences. And the problem at the moment is that it's relatively hard for those people to, you know, switch fields. That, that, you know, once you've done a few post years of postdoc, you tend to be particularly in the academic community, you tend to be rather, you know, typecast, you are a string theorist, or you are a, you know, and if people leave the field, or want to leave the field, they're often perceived, oh, well, they must be, you know, they must be doing this cause they failed in their original field. They're just desperate to find something else as a sort of second best.
But that's not the way; that's not my experience. It's certainly not my personal experience. And I'm finding it now a lot with the sort of postdocs who apply for positions in my group, in Oxford, that they genuinely are motivated at this stage, you know, in there, you know, could be mid to late twenties or something, or even early thirties. They're now motivated to, they had a successful career, but they're motivated to do something that's going to be a bit more useful for their fellow human being.
And we need somehow, a system that can accommodate that type of change. You know, these days we're all living longer. We have productive lives much longer, you know, it's no longer the case that you are, you are burnt out as a theoretical physicist at age 30 or something. We all have productive lives much later in life. And there's no reason why we shouldn't be able to change fields later in life.
I have to say I'm on the, one of the committees that the Eric Schmitz organization, runs to actually fund this type of people changing fields late after Ph.D. And I think this is a great scheme, but it needs to be broadened more generally. And I think research councils and agencies and things, you know, around the world need to be taking this into account. There's an enormous pool of talent, in, in very sort of theoretical subjects that are, that are really keen to apply their techniques to, for example, environmental science. But they, we need to be able to give them a year of, you know, to retrain, to learn some of the jargon and that sort of thing for them to be productive than in research grants. So, I just say that's my little, my little be in my bonnet about this sort of stuff.
Steve Hsu: I completely agree with that. I think, a little bit later in life is the right place to focus our efforts. So, people who are post Ph.D. or post one postdoc or something who want to transition, setting up, you know, maybe five-year fellowships for those people so that they can really make a go of some other discipline, I think would pay off enormously in the long run.
Tim Palmer: Yeah, that's right.
Corey Washington: Don't you think this is a general trend? That, you know, a lot of people were interested in physics and mathematics, when they were younger, at least 30 years ago is a very trendy sort of status-driven to be focused on very theoretical questions. But it seems like the new generation is much more practical than perhaps we were initially. So, you know, I think back to my mathematics education, and I took almost, I took no prob statistic, no probability. Took a lot of very abstract mathematics that basically proved not to be useful to me or anyone else. If I could do this again, I would focus much more on applied mathematics and statistics, which I think many people realize has wide application.
Tim Palmer: Yeah. I understand what you're saying, but you're saying that with the hindsight of, of kind of adulthood and maturity and things. And I, I think that It's still the case that the very brightest, you know, undergrads and things, as they come to think about what they want to do for PhDs will go for those, let's say more abstract, you know, theoretical stuff because it is, you know, I mean, it is unquestionably fascinating. But the question really, as I say, that faces you in a very kind of existential way once you've come to the end of your Ph.D., and you've got a little bit of that, you know, you, you, you've had a crack at those sort of abstract problems is, is this what I want to be doing for the rest of my life? And I think that's where people today realize. You know, in the old days, those people, you know, they might go into defense, or they go to the, you know, to Wall Street or some bank or other, but people are saying well, realizing that that's not great, neither of those are great alternatives either.
And, and suddenly the whole environmental issue is, is hitting them in the face. And they think, well, God, I could, I can apply my talents in this area. So, I think that's what's new. I'm not sure that it's, that young people are necessarily thinking at an earlier age about practical issues. I, I, I just think that, I mean, although there may be some of that, but I, I think, you know, we're beginning to realize we are not typecast. We don't have to, you know, our career is not necessarily set in stone by doing a Ph.D. I think that's, that's where we got to, got to think differently if you like.
Steve Hsu: So, let me turn to the main topic that we wanted to discuss. You recently wrote a paper Tim, which was published in the Proceedings of the National Academy of Sciences and the title of the paper is the “Scientific Challenge of Understanding and Estimating Climate Change.”
So obviously because of its central importance, climate change has been a topic we've discussed before on this podcast. One of the issues that we've really wanted to drill down, or at least I maybe Corey's bored by this, but I really wanted to drill down on is, is really what is the level of model uncertainty, in climate change? How good are we actually at understanding the impact of greenhouse gasses, et cetera, on the long-term evolution of the climate? And your paper caught my eye because it seemed to really be focused on these issues. What, is the current level of uncertainty in the existing models? What do we really need to do to get to a higher level of certainty? And also, what are the key mechanisms inside the key physical phenomena that make it really difficult to model climate?
And I was hoping to kind of get into a discussion of that. not really for, you know, don't assume everybody listening is a physicist or a climate scientist, but I think by and large, our listeners are pretty technically sophisticated.
So, you don't have to pull your punches too much. But I would like to get into that discussion.
Sure.
So, let me start, by just reading; I think this comes from the introduction to your paper and it'll just kind of set the tone. So, you write that the idea that the science of climate change is largely settled, common among policymakers and environmentalists, but not among the climate science community, has congealed into the view that the outlines and dimensions of anthropogenic climate change are understood. And that incremental improvements to an application of the tools used to establish this outline are sufficient to provide society with the scientific basis for dealing with climate change. For certain, some things are settled.
And here's a list of things I think that you think are settled. We know that greenhouse gasses are accumulating in the atmosphere as a result of human activity and that they are largely responsible for warming of surface temperatures globally. We also are confident in our understanding as to why this warming is expected to be amplified over land masses and the Arctic. Likewise, we are confident in our understanding of how the hydrological cycle amplifies the effects of this warming and how warming amplifies the hydrological cycle.
For these and other broad-brush strokes of the climate change picture, we are increasingly confident in our ability to usefully bound the magnitude of the effects. From this certainty stems the conviction that additional warming is best avoided by reducing or reversing emissions of long-lived greenhouse gasses.
So, I was hoping that we could unpack that a little bit. So there are several observations in there of things that we should be very confident of, despite whatever uncertainties we're going to talk about later.
And so maybe you could go through those things for us.
Tim Palmer: Yeah. So, the, the opening paragraph, the opening paragraphs of the paper were trying to make it clear that although we are somewhat critical of the current state of climate modeling around the world, we are not in any way, you know, doing it from the perspective of somebody that wants to attack the basic science of climate change. So, we're just trying to lay that out pretty clear at the beginning.
So, what do I mean by that? We, we, you know, there's no question, as you say that greenhouse gasses are increasing, greenhouse gas concentrations are increasing due to our emission of fossil carbon. And we're, we have no doubt that that is causing a warming of the planet and that much of the warming that we've seen over the last century or so can be attributed to those emissions of greenhouse gasses. And indeed, certain aspects of the water cycle can amplify the direct effect of increases in carbon dioxide and in particular, the so-called water vapor feedback. In other words, as the atmosphere warms, the air can comprise more water vapor molecules, gaseous water molecules, and those are an additional greenhouse gas. That's an additional greenhouse gas, which then amplifies the signal.
So, you know, if you say that the world has warmed and has it been caused by us? We would say unquestionably yes. But you know, society needs to know more than that. There are two important issues facing us. One is how bad, how warm will it get over the next hundred years, let's say, or to the end of this century. And secondly, what does it mean in terms of local weather? How does the, you know, how does the weather over the UK or the US or any other part of the world, how is that going to change as the, as the global temperature rises? And what I mean by that is in terms of, you know, storminess or drought or, you know, rainfall amounts, whatever. Intense windstorms and so on. How, how are the frequencies of these things going to change?
Now, we typically look to our models to our computer models, which are, you know, essentially our best way of applying the laws of physics to the problem of climate change. So, the computer models are, you know, mathematical representations of the laws of physics.
This is an important point, by the way, they're not just somehow, you know, kind of equations made up out of thin air. They are based on, for example, the fluid, fluid dynamic laws called the Navier-Stokes equations, which are representations of Newton's laws of motion, that sort of thing.
So, the models are our best way of, of, solving the laws of physics. And we use them to try to get at both of these questions, how bad global temperature will be, how much will global temperature increase by, you know, over the next a hundred years or so. And secondly, what is the manifestation of that warming and that climate change to the local weather patterns, right?
Steve Hsu: Yeah. I just wanted to unpack two, two points that are made in your opening paragraphs. And just try to maybe have you give some intuition to our listeners about them. So, one of them was this reinforcement between warming and what you call the hydrological cycle and vice versa. You may have already said that, but I just wanted to make sure I caught it that you're here. You're talking about water vapor. So, warming causes more water vapor to be in the atmosphere and water vapor itself is a kind of greenhouse gas. Is or
Tim Palmer: That's right. So there, there, yes, there are actually two, two elements to this. The, the one is what, what you just said, which is water vapor is a greenhouse gas. So as the atmosphere warms, it will be amplified by the presence of water in the atmosphere.
This is one of many so-called feedbacks, which we may come upon, which make predicting climate change difficult because it's not purely just a matter of how much the carbon dioxide warms the planet, or how much extra effects are going to amplify that. And water vapor is, is one such amplifier, but actually that's, that's something we there's very little uncertainty about because water vapor is, you know, the atmosphere, you know, unlike clouds, which we'll come to, which are sort of the liquid or ice form of water, water vapor is fairly uniformly distributed. It doesn't vary so enormously compared to clouds. So, we have a pretty good idea that the water vapor feedback is a positive one. But just before we come to the end of that question, there is a second point, which is that, as, as the, as the atmosphere holds more water vapor, so storms when they form. So, you know, the intensity of a storm is very frequently determined by how much water vapor condenses into liquid water and releases latent heat of, the water latent heat of release of latent heat from condensation of vapor into, into liquid form. You know, everyone's aware, I'm sure. You know, when a hurricane makes landfall and then goes in land, a lot of the, driving, water that drives the latent heat release, which drives the circulation patterns get shut off because the air is no longer being fed directly by such moist air from the sea.
Tim Palmer: So that's, that's a manifestation of how storms, the intensity of storms is very much determined by the amount of condensation of water vapor to liquid water. And basically, as a general comment that as the atmosphere warms and holds more water, these storms will become more intense because the amount of water available to condense into liquid water is larger.
And we've kind of seen that. I think, in terms of the statistics, you know, statistics of extreme weather events are very difficult if you take one particular location. But averaged over the whole globe, we see a tendency towards these sorts of storm systems becoming more intense. So that's consistent with this general notion that as the atmosphere holds more water than the intensity of storms that are driven by the condensation processes increases.
So that's a very general comment. But that doesn't tell you exactly, you know, what will happen to the weather over California or over, you know, Southern UK or something like that, which is a much more specific question.
Steve Hsu: There's a statement here about specifically knowing that the feedback effect is positive from water vapor and whether we know the sign of the totality of all the feedback.
So, clouds plus water vapor plus perhaps other things. Do we have confidence about what the overall size of these feedback affects our relative to each other and what the overall sign is?
Tim Palmer: No, we don't. And that, and that's one of the big problems that, when it comes to clouds, you don't have to look very far. And so, when you look at clouds, then, then it's a much more complicated problem. And one that we have estimates to solve, but I would say the estimates have big uncertainties in them.
So let me just say a little bit about clouds. So, clouds actually can have a kind of a jewel role. When you have very thick clouds, which just sit, I don't know, a kilometer or so above your head, or even, you know, lower, as everyone knows, they basically reflect the incoming solar radiation, the sunlight back to space. So, they're very reflective at the top of the cloud layer and they shield all that sunlight from the surface. So, the surface will typically be cooler when such clouds are around and when they're not.
On the other hand, if you take the very thin, what are called cirrus clouds, which exist at much higher altitudes, you know, 10 kilometers or more above the surface. They are typically much thinner, and they contain mostly ice crystal clouds, rather than water droplet clouds. And they play a role. It actually meant more like a greenhouse gas of trapping, the infrared heat whilst being relatively transparent to solar radiation.
Tim Palmer: So, a kind of worst-case scenario for climate change is that if these high-level clouds become more prevalent, and the low-level clouds dissipate, then you know, this is going to amplify the, you know, the basic climate change signal. If clouds do the opposite, the cloud amounts increase at low levels and decrease at high levels. The effect will be the opposite and clouds will, will be a kind of damping effect on climate change.
Now, most models actually suggest, unfortunately, that the cloud feedback is probably positive, which means the cloud, the way the clouds adjust to the warming signal is such as to amplify that warming further, which is bad news for society. But our view is that the uncertainties in these estimates are really, really big because we just cannot, even start to resolve the dynamics of clouds with the current generation of climate models. All we can really do is say that there is certainly a risk, a fairly such quantified well, and I'm not sure how easy it is to quantify it, but apparently a substantial risk based on current generation models that the cloud feedbacks are positive.
But, you know, it's, it's such a, in my view, it's such a, a kind of a fundamental question because it's almost this is, this is how our species will go. I mean, if cloud feedbacks are very substantially positive, then it really becomes climate change becomes an, an existential threat for, for large parts of society, particularly those living in, in, you know, parts of the world where temperatures can easily exceed, you know, 50 degrees Celsius and 95% humidity.
I mean, once you get up to temperatures like that, the human body just cannot physically exist anymore without going to an air-conditioned room. So, the only possibility is to migrate poleward.
Steve Hsu: So, Tim, I'd like to come back to the clouds in just a moment, but I want Corey to have an opportunity to get in here.
Corey Washington: Sure. I'd just like to go to the second level of your model, which is the water vapor effect. So, can you give us an idea of the magnitude of the effect of feedback on increased water vapor? How, what do we know about that?
Tim Palmer: Yeah, well, you know, I mean, if you, if you double carbon dioxide and only take the carbon dioxide only into account, then you're talking about a warming of about a degree or so for a doubling of carbon dioxide, one degree, Celsius one degree.
Yeah. The water vapor can double that. So, we're talking two degrees or so. And then the clouds on top of that can, can change it, can even double that again to four degrees or more. So that's, you know, these are, these are, substantial, effects.
You know, I mean, this is not, you know, this is not new physics. It was understood back in the 19th century, I think by John Tindel, that water vapor. In fact, in some ways, water vapor is the most important of all greenhouse gasses, a molecule for molecules, more important than carbon dioxide. But, of course, what we're doing with carbon dioxide is systematically increasing it by our emissions of fossil fuels. But the water vapor is kind of tagging on the back of that by increasing through that effect.
Steve Hsu: Would it be fair to say that uncertainty in the CO2 effect, say one degree Celsius per doubling, that uncertainty could be low, like 10% ish, uncertainty, water vapor. Maybe you could tell us what kind of fractional uncertainty in that number is. And in clouds you said it maybe could be even a hundred percent or 200% uncertainty in the
Tim Palmer: prediction.
Yeah, I think that's great. I, I'm not really aware that there's any substantial uncertainty in the carbon dioxide quantity because it's, well with one, with one proviso that, you know, when we do these climate projections, we have to assume that we know how the emissions of carbon dioxide will be changing over the, over the next, hundred years.
So, you know, the way to do that, the way that's typically done in these, IPCC assessment reports is, is that you, everybody runs the same, what are call scenarios, where the carbon dioxide increases at a certain level, you know, which would correspond to so-called business as usual, where we take no action to mitigate, or others where we, we invoke substantial, emissions cuts. But if you, but given, you know, given a scenario in a, in a prescribed scenario of how carbon dioxide is increasing with time, then the uncertainty and the direct radiative response to that increased carbon dioxide is tiny. I would, I think more like 1%, I would say rather than 10%.
When you're talking about water vapor, there is some uncertainty about how water vapor distributes as a function of latitude. But it's, again, not a very large number. Again, perhaps 10% is a ballpark. But you're right. Clouds are, a hundred percent, you know, we, we just don't know at the moment which direction the cloud feedback will go.
Tim Palmer: I have to say most models do say it's positive, but I'm. It's not easy. You can't solve the problem. This is an area where you can't just write down a simple back-of-the-envelope calculation and say, well, this is the way it should go. So, the models have to kind of follow this. We just don't know the mechanisms are so complex and interactive.
I just wanted to ask one more thing about these opening paragraphs that I found interesting. You say that warming is expected to be amplified over land masses and the Arctic. And I've read this in other climate papers, but I never understood whether is that an empirical result or is there a, is there in that case, a simple back-of-the-envelope argument that lets you know, that that would be the case.
Tim Palmer: Yeah, that's a, that's more of a simple back-of-the-envelope calculation. The, you know, most of the heat, when the sun shines over land is, you know, absorbed in the top, meter or so of the, of the, of the land surface. Very little diffuses down, at least on, you know, 2012-time scale you're talking about, but, but not so much diffuses downwards, over tens or hundreds of meters. But the ocean you've got circulates, which, you know, where warm water that's, you know, water that's been warmed at the surface then is abducted down below the surface and mixes, deep down.
So, so effectively, you know, the warming over land, the warming of the, of the air, if you like, or the warming of the surface, perhaps is better to way to put it over land is pretty much, you know, restricted to the top few meters, whereas in the ocean it's, it's, it's, it's, it's transported down over many potentially hundreds of meters or more. You know, if you mix a certain amount of heat over a big amount of mass you, the increase in temperature, the mean increased temperature will be smaller. So that's why the temperatures increase more over land and over the ocean. So, it's not a, I mean, all we're saying in that paper is it's not a profound statement to say that we understand why, you know, which, which indeed is what we see observation, why the warming is larger over the continents than over the oceans.
Corey Washington: Yeah. So, I have a question about the, again, water vapor, and I'm wondering is this increase going to begin at the surface of the earth? So overall it'll lead to higher humidity across the planet. And, if, so what's the, in what percent increased humidity are we looking at? And have people really looked at the effect of having higher humidity, not just higher temperatures on the effect of human survival, because I think everyone knows it's much harder to operate in, you know, 90% humidity than it is in 20%, at high temperatures.
Tim Palmer: A way that we can measure these things is through relative, you know, relative humidity. And what we're seeing is that as temperatures increase, the relative humidity of the air is staying roughly constant. So that means the absolute humidity is increasing at the same, if you like, rate as the temperatures increase to make the relative humidity constant. The warming effect, I should say, from increased water vapor arises where the, you know, the relative humidity increases through a, through a depth, through a column of the atmosphere in the, in the vertical. It, you know, if you just had relative humidity, only being larger at the surface, that actually wouldn't have such a big effect. It's, it's actually the fact that the humidity is, has increased right through the depth of the atmosphere.
But as far as the sort of physiological effect on humans is concerned, you're absolutely right. And there have actually been a few papers on this in recent years where people point out as you correctly say that it's not, you know, the effect on the human body is not just from temperature. It's a combination of temperature and humidity. And there's a thing called a Dew point temperature, which is sometimes used, which sort of combines temperature, and humidity in this way. So, you can say when the Dew point temperature exceeds some threshold whose value, I now forget exactly what that value is. 38 degrees or something that, you know, you, you get to the stage where, as I said before, you can get heat waves where the human body actually just can't lose heat. It means you can't sweat. You can't lose heat by perspiration. never mind direct sensible heat loss.
So that becomes, then that becomes, as I say, an existential threat to the, to human life. And we've had the, we've had situations like this in the last few years in parts of the world, particularly in the, in the middle east, I think parts of Iran and Iraq have experienced these sorts of conditions for a, for a few weeks, which are really very close to this combination of, of high temperature and high humidity where it's, it's impossible to lose heat.
You know, even sitting in the shade, you can't lose heat. And, you know, if this becomes more widespread around the world, which it certainly is projected to do, that becomes a real problem because what do you, what do people do then?
Steve Hsu: So, Tim, if let's suppose the cloud dynamics are very unfavorable to us. So, they yield a large positive feedback effect for climate change. Would we have seen that in the geologic record? So, would there have been times when the climate ran off due, you know, due to that effect in other things, and would we know about it?
Tim Palmer: I mean, you know, this is, I'm not, I'm not a, a great expert in paleoclimate, but there are certainly periods of time where the temperature has risen enormously. and I, people certainly have speculated that this must be due to, you know, to the cloud, to positive cloud feedback occurring, because there's no other way to sort of understand how these enormous shifts in paleoclimate can occur.
And of course, the mother of all examples of, you know, positive feedback is Venus, which has this so-called runaway greenhouse effect, which, which, you can view as a, as a kind of a sort of the ultimate in positive feedbacks where you, you now got temperatures of hundreds of degrees, which obviously. No, I mean there's no. I don't think people are well, in fact, people aren't suggesting that the Earth is going to turn into a Venus exactly. But it just makes the point that really, to understand the greenhouse effect and to understand climate change, it's more than just understanding the kind of radiative effects of carbon dioxide.
And let me just bring this right up to date, because right now people are putting the final touches to what's called the sixth assessment of the IPCC, the Intergovernmental Panel on Climate Change. And I think the report will be released sometime next year. And, for the last few years modeling groups around the world have been doing, you know, projections with their latest versions of the models to, you know, to get the latest, estimates of global warming. And over the last few years, most models or no say a, a significant number of models have actually, revised some of their clouds, what are called parameterization. So, I come back to this point that the clouds are not represented. If you like explicitly in the models with the law, you know, the fluid dynamical equations they're represented by a somewhat simplified formula, which attempt to describe the effects of clouds and the interaction with the circulation patterns in a sort of simplified way.
Tim Palmer: Anyway, these, these models, there's been a kind of a fairly widespread revision of some of the particular details of how much certain types of clouds have, how, how much of the cloud is made of water and how much of the cloud is made of ice crystals. Now, the thing is, it turns out this is quite a subtle effect because most clouds, you know, the actual ambient temperature in the cloud is, is well below freezing, is well below zero degrees. But the cloud liquid water is often in, in supercooled water droplet form, and that it needs some, you know, aerosol impurities or whatever to, to change it into ice. And so, this question of what is the, the balance between, super cool water, droplets and ice droplets is quite a tricky one to represent correctly in these climate models.
But anyway, a lot of the climate models have undergone some revisions to this. These are what are called cloud microphysics schemes. And it seems that the ones that have undergone this revision in terms of containing more super cool water droplets than previously are now producing much bigger cloud feedback effects, which is a kind of complicated thing to understand, but people are trying to do that. But the point is that a lot of these models now are producing, amounts of globe amounts of warming to a doubling of carbon dioxide that were not seen in any of the models that occurred in the previous IPCC report. So, we're going to find in the sixth assessment report, a number of models where the projected increase to, to a doubling of carbon dioxide will be over five degrees, five and a half degrees or more. Whereas previously the maximum was only around four degrees or four and a half degrees.
So, this represents a considerable shift in this latest report to this warming, to this, you know, rather I would say, I mean, I hasten to use the word catastrophic, but it's kind of, you know, verging on a really serious, movement towards these, the warm end of the spectrum from, from our human emissions.
Steve Hsu: So, I think the viewpoint you take in the paper is that to really resolve this cloud microphysics we need a more granular grid size in simulations that maybe really even take into account now of your soak’s evolution in the atmosphere. Absent that, how confident can you be in say the sixth IPCC modeling, sixth version of the report modeling that gives this increase in temperature, increase per CO2 doubling?
Tim Palmer: Well, I, I don't know how, how to put a level of confidence on it. All I can say is it's our best attempt, at applying the science of climate change to the problem of climate change, you know, given our understanding of the science, which actually is pretty good.
You know, we, you know, because most of the physics of climate change, it's not all of the physics, but most of the physics is kind of 19th-century physics it's to do with thermodynamics and fluid dynamics and you know, stuff that's pretty well understood from a theoretical point of view. The problem is that we can't solve the equations as accurately as we would like.
So, you know, when the sixth assessment of IPCC comes out, I'll say, this is our best attempt with this, given our science and given the tools we have to do the science. But you know, my view is that this modeling issue is so important that we're not putting nearly enough in the way of resources to try to understand and be more confident about some of these feedback processes.
And, and I should say by the way that, you know, the models are not just used, I mean, you know, we've talked a lot in this interview so far about global temperatures, how much will global temperatures rise? And I'm saying the latest, you know, stuff is five degrees, which is a lot, but what affects people on the ground, is not global mean temperature it's, it's what the weather does. It's like, you know, how many fires you're going to get in California or in, in, in Australia? How many intense tropical cyclones are going to hit Mozambique or, or, you know, or the Caribbean or wherever? How many droughts are going to affect Somalia or, you know, other countries in the Sub-Saharan Sahel? How many windstorms are going to whack into Southern England or, or whatever it is, you know?
So, these are the practical questions. You know, the UK has had some terrible floods from severe winter storms and, you know, knowing how much money to invest in flood defenses, you know, because of climate change is important. Knowing how to manage the forests under climate change in California and Australia is important. So having better models is not just about having a better grasp on what the global mean temperature increase is going to be. It's actually having a much better handle on how the extreme weather events are going to evolve, at a regional level. And, and in a way, you know, I feel that is the crucial point because, you know, we've got to cut our emissions, anyway, but on top of that, we've got to think about how we invest in infrastructure at the regional level to, you know, adapt to things that are going to happen anyway. And how do we spend that money effectively? And we don't spend very much money actually on climate modeling. I mean, compared to what we spend on, you know, putting space, you know, instruments into space, either looking at the Earth or looking at the stars and things, you know, is a lot more than we spend on, on modeling and, and computing.
Steve Hsu: Yes. So, you know, the way I would say it is if you're making what are effectively trillion dollars or even existential societal decisions, investing a billion dollars on better simulations seems like a reasonable investment. And it's, as you say, it's even cheaper than gathering observational data on this problem.
Now I think if I'm, correct me if I'm wrong, the current grid size for these simulations is kind of on the order of 10 kilometers cubed. Is that fair?
Tim Palmer: No, that's not quite right. I mean, if you take a weather forecast model that runs out to, you know, just 10, you know, you have to do a 10-day forecast every day or something like that, then yes. About a 10-kilometer grid, or, or even actually a little bit less sometimes is, is what's possible. But most climate models tend to have rather coarse grids than that. In fact, you know, this is the problem that a lot of the models that go into IPCC are still almost kind of associated with university groups and they don't have a lot of resources, for models or, you know, computing and things.
So, they end up being closer to a hundred kilometers. So, you get quite a spectrum of resolutions. The best ones are maybe down to 20 kilometers, but many of them are up at, up to a hundred kilometers or so.
Steve Hsu: Right. So, imagine you have some very nonlinear Navier-Stokes evolution happening a hundred kilometers by a hundred kilometers by, I guess the height would also be something like 10 or 20 kilometers, right. That you have to model out too.
Tim Palmer: No, the height tends to be, because the atmosphere is a very thin layer, compared to, you know, if you, if you, you know, if you take the Earth and imagine the atmosphere as a, as a layer, it's, it's, it's a, it's just a kind of, it's a tiny amount of thickness compared to the, the radius of the earth.
So, models would have, you know, the best models actually would be in tens of meters of resolution, but the worst ones, maybe a kilometer or so.
Corey Washington: Okay. Tim part of my role as the audience ombudsperson is to flag terminology that people may not be familiar with. So, Navier-Stokes, can one of you please give us a simple description of?
Tim Palmer: Sure. Navier Navier-Stokes. I mean, this is I'm, I'm pronouncing this word Navier like a typical, typical Anglo person that I, I am, I mean, Claude Navier. Navier was a French scientist who, actually I think to be honest, he actually predated independently of George Stokes who was an Irish physicist. So, Navier I French just Stokes Irish.
They both basically wrote down an equation, which was a kind of a manifestation of Newton's second law of motion. So, Newton's second law of motion is four equals mass times acceleration. I mean, Newton, when he, when he, you know, when he wrote down those laws was thinking of applying it to a planet. So, you have a single solitary object, which, which, you, you apply the force of gravity to and, and the object accelerates under the effect of that gravity, according to force equals mass times acceleration. The problem with a fluid-like, the atmosphere or the ocean fluid can be a liquid or a gas, is that effectively you have an infinity of little particles that make up that fluid, you know, if you like atoms or something like that. So how do you, how do you represent Newton's laws of motion apply to that whole fluid when it comprises an infinity of particles and what, Sir Navier and Stokes, as I say independently realized was you could use the language of calculus and a thing called a partial differentiation to write down a single now.
Tim Palmer: So, this is a, this is a good question. Cause what you can do is you can write down a single equation, using this language of partial the partial differential calculus, which expresses Newton's second law ma force equals mass times acceleration for this infinity of, of little, tiny atoms that make up a fluid like a liquid or a, or a gas. So as an equation, it's incredibly beautiful. It's a beautiful piece of mathematics. And in fact, you know, whole, even to this day, groups, you know, in the applied mathematics departments, you know, try to understand the theoretical mathematical properties of this partial differential equation that we call the Navier-Stokes equation.
The problem with it is if you actually want to solve the equation to get numbers out, you've got to go back down and break it up into these, you know, little atoms. And that's where the problem comes in: when you have a computer of a certain size, you know, you're given a computer. So, solve the equations. The first thing you have to do is say, okay, how small can I break my fluid up into, into pieces if you like into, into these sort of ATIC pieces, which will allow me to, make a, a, a, a projection of the equation, say you say, I want to, you know, I want to, I want to run the model out for a hundred simulated years. You say, well, I can't, you know, if it takes me 10, 10, real years to make a hundred simulated years, I mean, then I'm going to be waiting 10 years to get any answers out. So that's no good. So, you say, well, okay, a hundred simulated years, I could maybe wait a month. I'd say, you know, that might be a kind of calculation people would say. I can't wait more than a month to get that a hundred simulated years. So that determines how big or how small, I should say how small your atoms are, the, the kind of numerical atoms that you, that you go back to, to actually solve the Navier-Stokes equations.
And that's where this, you know, that's when I say most, models, climate models have these grids of a hundred kilometers. It means that the, the kind of atoms, I mean, I, the word atom I'm putting in quotation marks because they're not real atoms, but they're just sort of, you know, it's a discretization of this fluid into chunk size by hundred by maybe one or two or in the vertical. That is the smallest they can go in order to produce a hundred simulated years in, in a month.
Steve Hsu: So, I guess to summarize that a little bit, so you have these nonlinear partial differential equations, which describe the evolution of this fluid, correct. That we care a lot about. And, you know, solving these equations is remarkably hard. I even see papers where you might have a theorem that some mathematician actually proves about, you know, rigorous behavior of Navier-Stokes equations, that would be a new result, could be a new result. You could have a result where some people simulating on a supercomputer actually managed to reproduce some patterns that you see in your coffee cup. When you pour cream into the coffee, that's a hard problem.
So now imagine that I want to figure out what is the distribution of clouds, and ice crystals in the clouds and super cool droplets of liquid water in the clouds, up to an atmosphere of, I don't know, up to a, a height of, I don’t know, multiple kilometers at least, and I'm not able to do this with current computing power. And so, I actually subsume all of that complex physics, which is highly non-linear and it's chaotic and involves turbulence. I subsume that into some simple equation, which just says, hey, if the temperature and the pressure, and some other parameters within this huge cell, which is a hundred, maybe a hundred kilometers by a hundred kilometers, is this, then the distribution of clouds and the consequent reflectivity at the top and the infrared screening at the bottom, it is that. And, supposedly my little summary of all the complex stuff, in just these simple equations, is what turns out to be maybe the biggest uncertainty and the evolution of the bigger climate model. I think that that's the problem these people are confronting. Is that fair, Tim?
Tim Palmer: That, that, that, I think you sum that up reasonably well,
Corey Washington: Tim, so I want to come back to two points you've made. I actually, one question is we commonly say that climate is an existential risk, but of course it's relative to location. You know, I don't think that parts of Canada are going to suffer that catastrophically from even a five-degree temperature rise, but that's existential for Sudan or Iraq.
And I think this is related to your observation, that we don't invest a whole lot of money in climate modeling because perhaps the cynical autotomy says, look, the people with the money to invest aren't actually not facing existential risks. They're facing inconvenience. But the places that are really in danger of having the population wiped out don't have the money and are often complaining the loudest, but their desires aren't being heard.
So, what do you think about that? But first I'd like to hear your take on, you know, you talked about, we want to know the effect of weather in different parts of the world. Can you use a little bit of a survey, what you from your, your studies you think might have certain effects in different parts of the world, and then your reaction might take on, perhaps, what's motivating people to invest or not invest in models.
Tim Palmer: Well, look, can I, can I, can I attack, let me, I'll tell you some things about specific parts of the world, in a minute, but let me, because I have a sort of a slightly funny story about that. Well, you may not think it's funny, but anyway, I'll tell you anyway, but, but let me just first come on to this point about existentialism.
So, I think you're right that, you know, the citizens of Canada are probably not going to face existential threats directly from climate change, but I make two points. One is that sea-level rise will affect everybody. So, any, any low lying, you know, coastal cities. I don’t know whether Vancouver would count as one, but any low-lying coastal cities, faced with, with potentially meters of sea-level rise.
Again, by the way, that's another one of these massively unknown quantities, because we can calculate how much the sea level will rise directly from the thermal expansion of the water as it warms. What we can't figure out very well is whether the ice sheets over places like Greenland and, and parts of Antarctica will not so much melt, but will disintegrate. You know, it will actually dynamically collapse. We just don't know that. So that makes all the difference between sea level being, you know, a meter higher or several meters higher. And that makes a big difference for a lot of coastal cities.
The other point, which I want to make is, is I kind of maybe vaguely alluded to it. If you are, you know, bearing in mind that most of the population will rise over the next few decades in developing countries, large parts of Africa, and so on. If these people are facing, you know themselves, existential threats, then they are going to, as, as humanity has always done for, you know, thousands of years when the climate's got bad, you just move. You move somewhere where it's a little bit more equitable. And that basically will mean moving poleward.
So, you know, in Europe we've seen problems from migration, from, from countries facing, you know, increased climate threats. And I think in the US as well, you've seen something similar. I mean, this is probably nothing compared to what it could be like by the middle of this century. So again, you know, a mass massive uncontrolled migration poleward may not be an existential threat for the, you know, average, Canadian, but it's something which, you know, we, we need to start dealing with now rather than waiting for it to happen.
I would say at the moment, you know, this is trying to be confident about how climate change will manifest itself regionally is a problem that, again, we're a lot of uncertainties. But I remember some years ago I was being interviewed by, this is actually for a government position where there was a, a lady from the, the Scottish Devolved administration. And she asked me, well, what will climate change be like in Scotland? How will you know? Oh, no, there was no that's right. It wasn't so much, how will climate change? What were, what are the main health threats from climate change? Now, she was, I'm sure she was dying for me to say, oh, well, you know, there'll be massive incidents of skin cancer and heat stroke and all this sort of stuff, you know, as Scotland becomes this kind of new, new Mediterranean type of country.
Tim Palmer: But, you know, my experience is that actually, what will happen in a place like that is that the, and we were talking about this earlier, the increased humidity in the air will just make cloud cover for that part of the world relentless. So, it's bad enough in the winter when the daylight hours are very short anyway, of seeing the sunshine. My own view is that with climate change, you'll never see the bloody sun in the wintertime. And so, the biggest threat, I would say, health-wise is, is not, heat stroke and, and skin cancer, it's kind of depression, alcoholism, and drug abuse. And also, vitamin D deficiency, rickets, that sort of thing. Those are the big health threats.
So, all I'm saying here is that, you know, these things are very, very, subtle, issues and quite complex issues. And a big problem for the UK. I mean, my own experience is that we have had a number of very wet winters and there's a lot of money going into investment in, into, into, into flood defenses. But unfortunately, what I don't see, and I think people have sort of, haven't quite kind of realized this, that they think very much climate change is a, is a kind of a, a trend towards some, you know, in some direction like towards wetter, a wetter climate. But what we don't know is whether in fact the kind of extremes our climate will increase. So, it's not, you know, there'll be a trend, but there may also be an increase in the variance. So that, so the thing is what happens if there's an increase in winters or, or indeed whole seasons where high pressure, you know, anti-block, what are called blocking anticyclones form and persist, because then what will happen is we'll run out of water, will run out of potentially will have no renewable energy because the sun doesn't shine, the wind doesn't blow in a, in a wintertime anticyclone. And these are actually often situations where the air temperature can be quite cold. So, the demand for electricity can be quite high. So, you know, planning carefully for our energy needs in the future, you know, really requires us to answer these questions quite carefully about not just, is there a trend towards wetter winters, but what is the, what, what, how is the, how are the variance of these types of extremes going to change as well? And are we going to get more of these extreme high-pressure winters, which would have the opposite effect where we'd run out of water, we'd run out of renewable energy and stuff like that.
Anyway, all I'm saying is these are unbelievably complicated questions and answering it from a model that's just been written, you know, by a small group of people at university to answer a few kinds of hypothetical, very large scale, kinds of questions about the physics of climate change, isn't good enough. We need to be developing models, you know, putting massively more investment into them than we currently are if we're going to really answer these important policies in society, important questions.
Corey Washington: Tim, have you ever heard of Richard Weller?
Tim Palmer: The name is familiar, but just remind me.
Corey Washington: So, he's a dermatologist from Edinburg, but he's done a lot of research on the effect of sunlight on cardiovascular health. It looks like getting too little sunlight is a risk for cardiovascular health.
Tim Palmer: I can believe that.
Corey Washington: He's got a talk on YouTube, which you can find called why are Scots so sick.
Tim Palmer: Yeah.
Corey Washington: And so, it's generally known that rates of cardiovascular disease go up with latitude.
Tim Palmer: Yep.
Corey Washington: And a bunch of Scandinavian studies have supported this also. So, I think one of the consequences of a very, very cloudy Scotland is even higher. Scots already have the highest rates of cardiovascular disease in the UK. Yeah. And even higher rates as a result.
Tim Palmer: Yeah. It could get worse. I mean, this is the sort of thing that could get worse, and people aren't, you know, thinking on those lines, they're always thinking of climate change as something, oh, we're going, if it's warmer, it must be, you know, we're going to lots of sunny, sunny days and sunny skies and everything, but doesn't necessarily work like that.
Steve Hsu: Yeah, to your question though, Corey, I mean, it is true that maybe in the global north or the economic north, it isn't an existential problem or maybe that most of the risk is not existential, it's more economic. But still, the amount of dollars or pounds that are at stake is so high that the amount of investment in sort of better computing to try to get better answers is, is it kind of criminal, how little investment it's equivalent to us knowing that a viral pandemic was a possibility. And we've been talking about it for 20, 30 years. Yeah. And then when the thing actually arrives, we're totally unprepared for it. So, I think it's that hopefully people will learn from the crisis that we're currently in.
Tim Palmer: No, I mean, I often say it's a, it's a matter of investing a billion dollars or so, which, you know, a billion sounds a lot, but on the other hand, a billion is what you spend on a single space, or something like that.
Steve Hsu: Or even a small particle collider. Actually we,
Tim Palmer: Yeah. I mean, it's, it's one, it's one. was it a year's worth of, of a, of a certain budget or something. Just, just finish off this point about, you know, I make the analogy sometimes with the Marshall plan, you know, after the second World War that the US, basically, you know, kickstart the European economy after the second world war through this massive injection of cash called the Marshall plan.
And, you know, I'm sure a lot of people like to think of it and I'm sure it was to some extent, an altruistic gesture. But everybody recognizes that it, that, you know, the US had an ulterior motive, which was to prevent Europe from becoming a communist continent. You know, it was a way to, you know, get the capitalist system working again and, and to prevent communism taking over.
I see, you know, trying to understand climate change in the developing world and to maybe, invest in the sorts of infrastructures that will make society there more resilient to changes in extreme weather, and that would include better, better, warning, you know, early warning systems, you know, to be a kind of a part of a modern-day Marshall plan.
And, and what you're trying to stop now is a kind of, as I say, an uncontrolled migration of people from these places by, say, the mid-century, because it's just become unbearable there. So, I think there's a good parallel with what happened after World War II that's worth contemplating.
Corey Washington: You know, I'm, I'm, Steve knows this quite well. I'm a little cynical, but human ability to react in the absence of crises. And so, we're seeing it right now with the pandemic, you know, we're putting in, you know, what's going to cost us a couple trillion, probably over the course of this. And right it could have been mitigated, definitely with a billion or so of investment.
But I think, you know, you are seeing much more discussion of these topics as you see migration pressures. But it's really an open question what it'll take to move policymakers, how much is the crisis of the border? not a great history World War two had to happen. And then you had the Marshall plan. Yeah,
Tim Palmer: No, I totally agree with what you're saying, but, nevertheless, we have to say it.
Steve Hsu: Yeah. So, Tim, are there large funding sources that are becoming available for this kind of simulation? Are you optimistic about where we'll be said 10 years from now in terms of the quality of climate models?
Tim Palmer: I mean, in Europe we have the European Union and, you know, a group of us, we have a, we have a, a project called ExtremeEarth, which I would encourage any of your listeners to check into the website, extreme earth.eu, I think it is or something like that. Where we are trying to engage with the European Union to fund this type of project in Europe. You know, climate adaptation is definitely a high-priority item now in the EU. So, I'm moderately hopeful that something like that will happen.
I mean, I'm in the unfortunate situation of now belonging to a country, which is leaving the EU, which is not helpful very much. I'm in the slightly fortunate situation where my mother was Irish. So, I actually have an Irish passport as well as a British passport. So, if the worst comes to worst, I'll move to Dublin and set up shop there and carry on with my EU connections.
Tim Palmer: But we're waiting to see how the EU negotiates, sorry, how well, how the UK and the EU negotiate. I mean, there is a chance if things work out well, that we'll end up like Switzerland or Norway, which is, we're not formally part of the EU, but we still have all kinds of scientific connections to the EU through these framework programs and now horizon Europe programs.
Steve Hsu: Well, I'd like to tell you what sort of hopeful or optimistic story from my own 30-odd years in physics, there's a simulation project called Lattice QCD, which is this incredibly difficult computational problem of simulating the dynamics of quirks and gluons inside of protons and neutrons and nuclei. And this is a super nonlinear system. It's actually quantum highly quantum mechanical, but the basic equations are known. The basic equations are almost as simple in a way as Navier-Stokes equations, but, but take incredible resources to solve.
When I entered the field, there was no hope that we would ever solve and derive from first principles the properties of protons and neutrons and other strongly interacting nuclear particles from the properties of quarks and gluons. But over 30 years through just incredible effort, both theoretical effort and also just better and better compute, we finally can extract, precise predictions about things like I know nuclear, new electric [unclear] moment of the neutron or mass of the proton or the pion from first principles from quark level stuff.
So, you know, it's one of these things where if you look at a cloud directly, it doesn't seem to be changing very much. If you look away and look back again, the cloud has changed tremendously. And in the case of Lattice QCD, that's what happened. These guys worked with this distributed group of people all around the world, worked on this problem for 30 years and eventually kind of solved it. And I think the same thing could happen with climate. So, you should just keep up hope and keep pushing, even though the problem itself is super hard.
Tim Palmer: Yeah. I agree with that. And I, I think there is hope.
Let me, um, let me make a comment that's maybe slightly more relevant to the US, which is that, you know, I think we have actually achieved some remarkable things in Europe through the European Union. And, essentially what it's meant is that, you know, countries of Europe have come together and kind of pooled resources and done things that individual countries could not have. And I think there's a parallel here, in the US, at least from my field of climate that, there are all these federal agencies like, NOAA, the national oceanographic and atmospheric administration, NSF, DOE, NASA, department of defense and so on, they all have their own kind of semi-independent capabilities in this climate area.
NASA has a climate model because it likes to sort of compare them, observing space observations with simulations. NOAA has stuff to do weather forecasting and, and NSF has labs in Boulder that do, you know, climate prediction things. DOE have their own climate models.
I think, to make progress in this area, this is a problem where the agency should actually come together. And say, we can actually do better if we join forces and create some, some new generation climate models where, you know, maybe different agencies focus on different aspects of the problem rather than trying to invent the wheel each time themselves, which I think has been kind of wasteful of resources.
I think it would be better to, to actually join together and, and have a, you know, a concerted effort in, in, in America on, on solving this problem. I think that would really advance the science massively that happened.
Steve Hsu: I agree with you. I, I think probably the problem, the problem is just this kind of, interagency, bureaucratic friction or competition, and that prevents this kind of thing from happening. But I agree with you. If they pooled their resources, they would get much more bang for their buck.
Tim Palmer: Absolutely. Yeah.
It's, it's really been a pleasure talking to you, Tim. And I, I think our listeners will have a much better feel actually for the actual scientific problem of modeling climate after listening to this.
Tim Palmer: Well, it's been a pleasure to talk to you and, you know, hope we uh, come back in a few years and we'll see how, how things have progressed hopefully in the right direction.
Steve Hsu: That would be great. Thanks a lot for your time.
Tim Palmer: Okay.
Corey Washington: Yeah. Thank you, Tim.
Creators and Guests
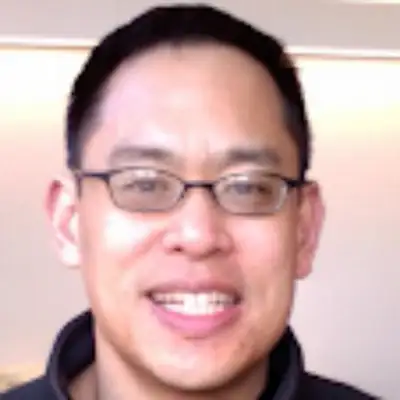