Michael Kauffman on Cancer, Drug Development and Market Capitalism – #48
Corey: Our guest today is Michael Kauffman, Co-Founder and CEO of Karyopharm Therapeutics, which develops cancer drugs for the treatment of a variety of cancers, and has an approved drug Xpovio for resistant myeloma. The company is testing low doses of Xpovio in patients with severe COVID-19. Prior to Karyopharm, Michael was the Chief Medical Officer of Onyx Pharmaceuticals Incorporated, which acquired Proteolix Incorporated, where he led the development of Carfilzomib, a novel proteasome inhibitor.
Corey: In 2002, he became CEO of Predix Pharmaceuticals, which was acquired by EPIX Pharmaceuticals in 2006. Michael led the combined company until 2008 when it went out of business after a failed phase three clinical trial in major depression. Prior to EPIX, he was leader of the Velcade development program at Millennium Pharmaceuticals, and held a number of senior positions at Millennium Predicted Medicine and Biogen. Just as the web was exploding in 1994, Michael created one of the first online medical textbooks. Michael received his MD and PhD from Johns Hopkins Medical School, and is board certified in internal medicine. Welcome to Manifold, Michael,
Michael: Thank you very much, Corey. It’s great to be here.
Corey: I have to say that when COVID hit, and we began thinking about possible guests to have on the show, to discuss issues related to the pandemic, you’re one of the first people I thought of, but I knew your cancer guy. I had a sense you might have something up your sleeve when it comes to COVID. And after getting in touch with you turned out that I was right. So, I want to go back to your background because I think it’s pretty interesting. And, our readers have a sense of what it’s like perhaps to start companies in tech, but don’t think our reader, our listenership is very well informed about what’s likely to start up some biotech.
Corey: So, I want to start with your current company and then backtrack if we could, and talk a little bit about, how you started previous companies and how you got to the point where you’re at. What does Karyofarm do?
Michael: So, Karyofarm was started initially by my wife, who had left her previous company, and wanted to come up with something that approached cancer from an entirely new way. And it was applicable not to one type of cancer, but potentially to many types of cancer. So, she began reading the medical and scientific literature. And, after reading a lot of stuff and coming up with four or five ideas, all of which I killed. She came up with this idea to attack the protein export and one, which was from a rapidly emerging new field of nuclear cytoplasmic transport in the cell, and seemed to be relevant across many cancers. And her idea was to create new small molecule drugs that would block this protein, and could inhibit the growth of cancer cells specifically without really hurting normal cells.
Corey: So, there’s a lot in that statement Michael. Our listenership is very sophisticated, and we have a lot of scientists. And our general approach is that we don’t simplify things. We state things as precisely as they can be scientifically. And then we explain in detail some of the concepts. So, let’s begin with cancer as a disease to treat. Why is it so hard to treat cancer, and give us an idea of how you came to approach cancer, the way your wife came up with?
Michael: Yeah. So, obviously there are many different types of cancer, but what all cancers have in common is that they’re uncontrolled proliferation growth of different cell types. And depending on where the cancer started, you get different cell types. But, basically the cells behave in a way that is focused only on their own existence and not on being part of a multi-cellular, multi-system organism. And what we know from cancer is sort of the pillars of hallmarks of cancer, if you will. And this is Steve Weinberg’s conception of it.
Michael: And one of those major pillars of cancer is that there are certain alterations of our normal cell processes. There are about six different major pillars. And cancer is really tough to treat is because all of these six alterations are actually pretty similar to what we do in the normal circumstances. And in particular, cancer has co-opted a lot of the different functions that we use during stress responses, that we use during infections, that we use during appropriate inflammatory responses, wound healing, and even embryogenesis.
Michael: And when you start to interfere with the processes of cancer, you end up interfering with normal cell processes, and ending up with the kinds of side effects that we get. It’s very different from treating other diseases, especially infections, where you’re dealing with a completely unusual different agent. And in fact, the farther away from us those agents are in terms of evolution, the easier it is to treat them typically.
Michael: We have some really good antiviral agents. We’ve cured Hepatitis C virus. We have great anti HIV drugs. We have decent drugs against the herpes viruses and so on. We can treat most bacterial infections. In fact, we can treat almost all bacterial infections. And the predictions of total disaster with bacteria have largely not come through because we’re really good at coming up with novel ways to treat them. We’re a little less good at treating parasites and fungi, mostly because those types of cells are eukaryotic in nature as opposed to prokaryotic or viruses. And, they’re just closer to us.
Michael: Cancer represents a disease that is so cloned that normal cells do, that coming up with truly insightful ways to treat it, requires a lot more clever approaches and is more subtle if you will than treating infectious diseases.
Corey: I think most people are familiar with the idea that cancers result from genetic mutations that cause cells to reproduce uncontrollably. Now, when you said there’s six different ways in which cancers develop, you mean six different classes of mutations, is that a proper way of thinking about it? Or six different.. How would you connect the general sense of cancer is a result of genetic mutation leading to runaway cell growth with the six different forms of cancer or at least origins that you mentioned?
Michael: They’re usually characterized as hallmarks and it’s a little bit, this is us applying our own sort of classification systems to things that evolved over a long time. But the genetic mutations are involved essentially in all six of these different hallmarks of cancers. One of the main hallmarks is mutations in genes that control growth in the sense that they drive growth. So, growth driving genes, which are very appropriate when you have a wound that has to heal. You better make sure that cells are able to divide, and create new cells to cover over the wound and take care of things.
Michael: It’s the same thing when you have a broken bone, when we get into trouble with various organ injuries, recovering and so on. So, those kinds of proteins, when they are mutated end up leading to uncontrolled growth under a cancerous situation, whereas under normal circumstances, they do things that are very important. It turns out a lot of those same proteins, proteins like Smac and the Src family of kinases and so on and so forth. All of these proteins are important in embryogenesis. That’s how we get from being a two celled fertilized egg into becoming a multicellular organism.
Michael: These are the a lot of the same proteins that drive the rapid growth and development leading to eventually in most cases, a normal birth after nine months of pregnancy. You need all that stuff. Now that’s one aspect of mutation that occurs in cancer and it’s bad.
Corey: So, it just hijacks these natural cellular processes in some way?
Michael: That’s right. It turns them on forever. So, there’s no way to turn them off.
Corey: Got it. Can you give us an example of… Let’s take myeloma you’re focusing on. What’s a typical pathway for my myeloma to develop from a normal cellular process.
Michael: Right? Well, so for myeloma itself, there’s actually a lot known about the normal side of the processes that are co-opted. One of them has to do with these kinds of changes. For instance, Bcl-2, the Bcl-2 so called oncogenes. Bcl-2 was named for B cell lymphoma, second gene that was important in its genesis. But it turns out to be important in some forms of myeloma. And when you mutate that gene, you end up with a set of plasma cells. That is the cells that produce antibodies in your body that become myeloma.
Michael: They become cancerous plasma cells. And in mutation in this gene seems to drive that particular form of myeloma. Now there’s another form of myeloma, a subtype that involves a separate set of genes. And those are called tumor suppressor genes. We have about 20 different tumor suppressor genes who are named because they do what the name says, which is when your body has a mutation that leads to cancer, we have a bunch of genes whose job it is to prevent that cancer from ever really growing.
Michael: So, all of us every day come up with cancerous mutations. This is a normal process, it’s part of our DNA replication. We have three billion base pairs in our genomes every time our cell divides, it makes 500 or a thousand errors in that division. Some of those errors would be cancer causing, but we never get cancer most of us. And the reason for that is we believe is because there are about 20 different genes, and they code for proteins whose job it is to make sure that we don’t develop cancer. Those tumor suppressor proteins act as auditors’ for our cellular DNA.
Michael: So, when you’re having mutation in DNA and the genes change, you have another set of orders or placement If you will, whose job it is to detect the changes, and either repair the changes, or if there are too many bad changes to tell that cell to commit suicide. These are tumor suppressor proteins. The best known is p53. It’s also called the guardian of the genome, and it literally guards your genome. Now, one of the funniest realizations or one of the most obvious ones now, realizations that Dr. Sharon Shacham, my wife, who founded the company had was that, in order for these tumor suppressor proteins to work, they have to be in the cell nucleus.
Michael: And, this was known. But, when I say it was known, it was one of those facts that was around, but nobody really talked about it. The reason these tumor suppressor proteins have to be in the nucleus is because they’re looking at the DNA. And that’s where the DNA is. Cancer cells have figured out that if you just transport these tumor suppressors out of the nucleus, they stopped working. And what Sharon realized was that there’s a single transporter for all 20 of these major tumor suppressors.
Corey: So, Michael, one question. If these proteins have to be in the nucleus to function, why would they ever be transported at the nucleus in the first place?
Michael: Well, in order to do the audit, and this gets a little bit of an analogy, but I think it actually works. When you have your books audited, if you’re having your accounting books audited, you have to stop the transactions. You can’t go in and audit something while there’s active transactions going on. So you freeze the books if you will. The same thing the cell does, in order for the cells DNA to be audited, it’s cell cycle has to stop. So, every time the cells undergo this audit process, they come to a halt, their replication stops, and they are audited.
Michael: If you ever want them to start replicating again, and in fact doing many of the normal things that they do, you have to release this block. Tumor suppressor proteins by their nature, induce cell stoppage. They block the cell cycle. That’s a normal process, so that the audit can be carried out. If you never let these tumor suppressors out of the nucleus again, the cell will never be able to divide again, and we’ll stop doing the things that it needs to do. And, the belief is that you can’t have one without the other. If you’re going to have an audit, you can’t be doing your normal cell functions, a normal cell division.
Corey: So, describe Sharon’s realization then. She found a drug that kept these proteins in the nucleus?
Michael: Exactly by blocking so, so what her eureka moment, and it’s one of the simple elegant solutions that you say it’s either really right or really wrong, and we can test it. And she said to me, well, every time I read about one of these tumors suppressors like p53, BRCA1, the gene that’s involved in hereditary breast cancer and so on, p21, p27 a lot of these proteins. It turns out all of these tumor suppressor proteins are chaperoned or escorted out of the nucleus by a single carrier molecule called exports in one.
Michael: Now that may sound obvious and maybe by known a little bit. But, when you realize that there are eight different export chaperones, when biology decided that one and only one protein should be in charge of exporting tumor suppressors, as well as a bunch of other proteins. But the fact that all 20 tumor suppressor proteins are escorted by the same protein out, suggested that there were some deep biology here. And what she realized is if you could design a drug that absolutely specifically inhibited XPO1 only, and none of the other seven transporters and none of the other important proteins with the cell, then we would have a chance to restore these tumor suppressors into the nucleus.
Michael: In cancer cells, they would look at the DNA and figure out that these cells should commit suicide. In normal cells. The tumor suppressor cells would go in the nucleus stop cell division, but realize that everything was fine. And when the drug went away, the export block goes away and those cells could continue. But again, back on the cancer cells, if we block nuclear export of tumor suppressors, the tumor suppressors accumulate in the nucleus, they look at the cell, they determine it’s cancerous, and they initiate cell suicide called apoptosis.
Corey: Once Sharon had this realization, what was the next step?
Michael: So, first thing to do was to try to prove it quietly, prior to any sort of patent filings or anything, we had to invest some money. We put about a half million dollars of our own money into it. And she went through her secret sauce as a drug discoverer, and her trainings in biophysical chemistry was to obtain the three dimensional structure of this protein, which ironically happened within about a month of her coming up with the idea. It was literally about March 2010, that she actually thought of this idea.
Michael: And in April, the 3D structure of export and one was published on the public databases by Human shotgun and some other people down at UT Southwestern, Human Chuck we’ve subsequently worked with a lot, but, she downloaded the coordinates of the protein. And she set off on her computer based algorithm. This is a combination of commercial software plus our secret sauce that she had developed in a previous company, in her PhD thesis, and looked for small molecule drugs, just standard chemicals that would inhibit this particular protein.
Michael: And this computer based algorithm really allows looking at 3 million compounds that are commercially available across the entire world, quickly on a computer, narrowing it down to literally 55 zero compounds, which we could purchase.
Corey: So, I want to get sense, Michael, this is purely simulation, right? You’ve got a sense of the 3D structure of these molecules, and you’re simulating their interaction with this transport molecule.
Michael: Exactly. This is like a lock and key. We think of drug discovery as, the protein represents the lock. And the drug is sort of the key in not to open the protein in this case, but to block it. But that’s the goal.
Corey: So, how can you tell this from simulation? What exactly were you visualizing? Not simply binding to the protein, but how could you tell it was blocking it?
Michael: So, that’s a great question. It turns out that most small molecules that bind to proteins, not all, but most turn out to be inhibitors. So, you’re quite right. All we were doing was actually looking for energy minimization regions where the energy of binding would be minimized, which would be maximal binding finding. We couldn’t tell a priori if these were inhibitors or activators of the protein, what happened then was she actually ordered 50 compounds as I mentioned, from different chemical companies.
Michael: We actually received them in our house. We stored them in our kitchen and our refrigerator literally. And we sent them off, we changed the name, she made codes. And, because you don’t want to tell the assaying company what you’re actually testing, because if it’s positive, you want to be able to move ahead now and file patents. So, we coded each of the chemicals sent them off to one of the standard places that do this. It turns out it was Thermo Fisher, a well known company, they were doing nuclear export assets.
Michael: And lo and behold, about eight of these compounds showed significant blockade of nuclear transport in the Thermo Fisher asset. And the best compound represented a starting point for the company.
Corey: What kind of cells are these components being tested in?
Michael: So, the initial test is interesting. It could be almost any type of cell. I believe it was an osteosarcoma cell line to begin with. But, importantly it was an osteosarcoma cell line that expresses high levels of the HIV Rev, Rev protein, which is known to be an interactor with XPO1. So-
Corey: Michael, stop for a second. Can we define a few of these terms?
Michael: Sure.
Corey: Osteosarcoma first, can you describe what cancer cell that is?
Michael: Yeah. Osteo is for bone? So, this is a bone tumor. Sarcoma means a tumor of connective tissue. So, this case it’s a bone connective tissue tumor. It’s a fairly uncommon tumor thankfully. But, it’s a cell line that can grow very easily in the lab. It grows fast, it grows easily. It’s just an easy workhorse for these kinds of assets. And, somebody had transduced a gene, actually one of our collaborators from Harvard medical school, pam silver.
Michael: One of the co-founders in our company had moved the gene for the HIV, the human immunodeficiency virus, Rev protein, Rev protein, which was known to be transported by XPO1. And in these cells, they had played around with it such that the cells express the rev protein only in the cytoplasm. But when you added an XPO one inhibitor, when you blocked a nuclear transport, the HIV Rev protein ended up in the nucleus.
Michael: And, that way you could tell if you had a positive compound. Now, the reason we actually able to know this assay works is because about 20 years ago, someone had discovered a bunch of natural products that actually inhibit XPO1. And these are products that are made by cell bacteria. So, it’s an interesting evolutionary story that these cell bacteria use XPO1 to fight other, pardon me, cell fungi, to fight other fungi.
Michael: And, it’s just evolutionary conserved.
Corey: So, somehow these other cell bacteria, sorry fungi attacking these cell in a way that gets their tumor suppressors out of the nucleus?
Michael: Well, it’s a little more subtle than that. I didn’t mean to make it more complicated,
Corey: Sure.
Michael: But, you asked me for the details. So, it’s a great story. We believe that evolution allow the development of these natural product inhibitors in order to prevent…
Michael: Inhibitors in order to prevent competing fungi, if you will, from taking over. So in nature, of course, there’s always fighting going on, there’s always selection, et cetera. And a set of fungi had figured out that if they produce this particular toxin called leptomycin B, that leptomycin B could kill other fungi that we’re trying to get … take over their space, if you will.
Michael: And this leptomycin B turns out to block export in one, and so natural product. It’s a nasty compound, it’s very toxic. People have done studies in animals before and they just makes the animals extremely sick or kills them. But leptomycin B serves as a nice tool in the laboratory to block this export one. The assay was developed by this Harvard researcher, Dr. Pam Silver, based on leptomycin B working in it. But no one had a drug yet, a real drug like compound that could actually block XPO1. And no one could really do any kind of cancer testing with leptomycin B because it’s just too toxic. It pretty much kills everything, But we had the assay.
Michael: So you asked earlier, how do you develop a company? Well, you need to figure out a target and the target needs to have an assay where you can tell that you have inhibitors or activators of the target as the first step, and Sharon was able to do that. She was able to take the three million compounds from the world’s vast array of chemical libraries. By the way, this is the theory because you get it all on the computer. It’s all downloaded catalogs. It’s a lot cheaper than doing robotics and actual wet assays, one at a time. And she honed it down to 50 compounds of which eight actually worked, and the best one inhibited or blocked XPO1 transport somewhere around two or three micromolar, which means it took two or three micromolar to inhibit transport by about half.
Corey: So give me some scale, Michael, two, three micromolar, give me a benchmark. Is that a lot, is that a little?
Michael: Yeah.
Corey: Compared to what?
Michael: That’s a great starting point. Thanks. We take it for granted, the drug development industry, but anything that’s lower than 10 micromolar is considered a good start. Most drugs that block targets, especially in cancer, do so in the nanomolar range. So we had to improve things by about a thousand fold before we could get serious and start to go into significant animal testing and then human testing. But as a starting point, two to three micromolar was a very good starting point. And what we knew is we had to modify that, calm those chemicals then to do better. To get it down into the 10 to 20 nanomolar range was the goal.
Corey: Got it. But before we hop in more, so we have a division of labor on this show. One of us tends to go into detail with our guests, the other’s the audience arm bits person. So I guess Steve, any questions at this point for Michael?
Steve: Yeah. I have a couple of things that maybe the audience would like to hear about. One, this development, it sounds like, I think you mentioned storing things in your home. Was it not also covered under the university IP for these researchers? Was it done partially at university?
Michael: No. Actually, the assay itself was never patented, which was interesting. Although Thermo Fisher sold it and it was available to anybody. The chemical compounds were available through catalogs throughout the world. And you cannot patent, at least not composition of matter patents, meaning you can’t patent chemical structures that already exist. You can attempt to patent new uses of those chemical compounds that already exist, but in pharmaceuticals we generally try to come up with completely novel compounds.
Michael: Now, we always start with … we often start with existing compounds that are known, but we modify them to such an extent that it’s not obvious that you would get to a drug. So no, there were no actual patents in all this. And part of our early work when we started to have to get lawyers involved was to make sure that there were no competing patents and nothing we had missed.
Steve: So this research, I guess, it sounds like a big chunk of it was just mainly informatical. Like looking at structures of molecules and doing some computer matching. Was it really done in someone’s house, like on someone’s Mac at home, or was there more of a laboratory infrastructure, but not at a university that was being utilized?
Michael: No, this was truly an at home effort. As I like to say, the public records would show, we didn’t have any offices. I was the chief medical officer at the time at proteomics and NaNotics as Corey mentioned at the beginning. So I was running back and forth to San Francisco, we lived near Boston in Newton. And Sharon was working on this literally at our house on a PC. I don’t think Mac could run the software back then, but I think they can now. And it’s remarkable that what used to take a series of like 24 Sun Microsystems computers in parallel could be done on a laptop for these rather extensive binding curves or stimulations. We ordered the compounds and then we coded them at home, sent them off to Fisher Scientific and got the Thermo Fisher and got the results. This was all done before we ever had any office space.
Steve: This sounds like a story where advancing compute technology and these databases that are publicly available are accelerating drug discovery. I always hear this story that the pace of drug discovery has really slowed down a lot of the big public companies, their pipelines are not what they used to be. Can you give us a better feeling for what’s actually happening in the world of drug discovery right now?
Michael: Yeah. The problem is not so much that we can’t discover the drugs. The drugs are actually fairly linearly … it’s fairly easy to discover new chemicals. The problem has really been target identification. The key for all human diseases is figuring out what the right target or targets are to interfere with, either to block them or to augment them. And coming up with what we call biologically validated or physiologically validated targets for diseases is difficult. And that’s been the real tough part of all of medicine. A lot of the chemistry has been solved. We can make many compounds that even we couldn’t make before, but finding the right biological targets has been a tough thing.
Steve: I’m not sure I understand that. This biological target step, is that something you have to do in mouse or something? Is that ahead of you guys or is ahead of the story that we’re at right now?
Michael: Yeah, let’s take a step back. It’s a great question. There are about 30 … we believe there are about 30,000 human genes and making about 30 to 60,000 proteins. And you can argue about definition of proteins that are highly related and identical or close enough and so on. But there are somewhere around 30 to 60,000 proteins in your body. The goal of most medicines is to block one or two or three or four of them, or to augment one or two or three or four of those proteins. The trick is to find out what the right protein is to block.
Michael: I’ll give a simple example. For HIV, we have over 30 drugs now I believe approved for HIV, and we have five or six different classes of drugs that attack HIV because they attack different parts of the HIV virus. Usually you give three of the drugs at one time, and that gives really good results, and it suppresses virus usually for many, many years. And when it stops working, you change to attack different other proteins in the HIV.
Michael: Well, human diseases are a lot more complicated, right? HIV, we know the genome, we know all the different proteins involved in HIV, at least for the virus. But in most human diseases, we don’t know all the proteins that are actually involved in the pathogenesis or the development of these diseases. So we have to figure that out in two ways. One is we do all kinds of genetic manipulation on model systems. This gets published and you can read almost all these papers in Medline and so on. And there are many good colloquium and seminars and big meetings where we discuss new targets.
Michael: So new target identification is usually done biologically with many different techniques, siRNA and so on and so forth. There’s just a lot of ways to try to figure out new targets. The problem is there’s no good model system really for humans except for humans. And the only real way to test most new drug targets is to make a drug that can go into humans. And actually put it into people and hope that your animal model and your test tube model, if you will, actually was relevant. The second part of our target validation really depends literally on pharmaceutical products. We don’t know that … we didn’t know, for example, that erythropoietin was important for the production of new red blood cells.
Michael: We thought so because in animal models it is, but until we made synthetic erythropoietin and injected it into people and saw that their blood counts went up, we didn’t know that human would respond to erythropoietin. And this is the same thing over and over again. It’s one of the great difficulties in drug development. We have a lot of great ideas. We have a lot of great biological models systems that support those ideas. And you’ll often hear the cynical comment that we cured mouse cancer two decades ago, because mouse with cancer actually respond to different things that we do. And then we go and translate that into humans and only about 5% of the things that we think are going to work that worked in animals … they all worked in animals, but only about 5% of the things that worked in animals actually work in people.
Steve: That was a great explanation. Can I just back up for one second. This basic idea of finding the molecular target or say finding a protein that you want to affect, with 30,000 to work with, but a very complicated organism like a human, how often is it the case that you have a clue that to cripple HIV you need to affect a particular protein, but that drug has tremendous side effects on other functions in the organism and you just have to abandon it for that purpose. Is that a common occurrence, or can you just get away with attacking or suppressing certain proteins and it only does the job you want without doing other things?
Michael: Yeah. I know my infectious disease colleagues wouldn’t be thrilled with me. But I would say one of the reasons it’s easier to treat infectious diseases than it is cancer is because when you’re dealing with an entirely different organism, like a virus or a parasite or a fungus or bacteria, you know those organisms have proteins that are unique to those organisms and you can make drugs that target only those proteins in the offending organism.
Steve: Got it.
Michael: Now, many times the drugs that we make will, mistakenly will … and it’s the question you ask, “Are you sure that they’re working on the right thing?” But they also hit stuff you don’t want them to. And that’s the old case of, well, we made a great key and it opens that lock or it blocks that lock, but it also blocks a bunch of others that you didn’t want it to do. And again, we do animal testing and I believe we still need to continue to do that until we figure out a better way. We test our drugs on at least two different species before they ever get into humans because most of those compounds that are going to be toxic to humans are actually going to be toxic in animals also. Not all, but most.
Michael: And we can eliminate maybe 20 or 30% of compounds right away from ever even getting into humans by testing in animals. We also have a bunch of assays because we don’t really want to test things on animals. We’d prefer to do it in test tubes. We have a bunch of preliminary assays in test tubes that can allow us to predict when drugs are going to be toxic. Many drugs never even get into animals because they fail these toxicity tests in the test tube, then they go to animals. And then in humans, we still have somewhere around a 10 to 20% rate of drugs that end up being too toxic for humans and don’t do the desired thing and therefore we have to abandon them.
Steve: So just to emphasize a point that Michael made, which is that a cancer cell is actually a cell that’s operating in your body. And so to find a protein that interferes with some aspect of its function, it’s innately more dangerous than trying to do it to some invading virus cell or something, which is very different. I guess that was a good point to reinforce. In terms of the capital requirements to go from idea to actual drug, maybe you can describe for our audience, what do you need to do this preliminary level of testing? What do you need to actually get it into a trial where actually using humans? How does that look?
Steve: One of the things that shocks entrepreneurs from other areas of technology is that in the drug discovery business companies IPO at a very early stage, before they’re ready to really market a product or sell anything to anyone … and I guess it’s largely on the promise of what they’re going to develop. And so I think the capital requirements stream is just very different in your industry than say what people in software are used to.
Michael: Yeah. We unfortunately never find out if we have a product until the FDA blesses our product. And that’s typically anywhere between five and 10 years after it ever goes into people. Like you say, it really depends on selling an idea and people trying to read the tea leaves early on. You do have stages and there are stage gates and there’s different capital requirements as you get further along. Most drugs today cost … for the successes, the overall cost of most drugs today is somewhere around $2 billion before it actually … if you include all the failures, because in larger companies you have to do a lot of testing and you have to pay for the failures as well as the successes are the only things that pay for them. Smaller companies live or die on whether their drugs are successful or not.
Michael: We spent about half a billion dollars before our drug was approved. And the hope by all investors is that eventually this will produce more value than we obviously ate up in that process. And I think it’s fair to say that that’s true. Our evaluation today is at least our enterprise value. So if you take the total value of the company and subtract the cash we have on hand, we’re about a billion dollar company, so we approximately doubled the investment now. But the expectation is that someday we’ll be a much bigger company as our sales increase and so on. The reason people continue to invest in this business is because the successes will really pay for things. Now, if you back off a little bit, it’s a little different than software, of course, and tech, where you have to actually produce something that works, get somebody to buy it, and then you really start to attract investors.
Michael: What people do in our industry is they come up with good ideas. Sharon had a good idea. We didn’t have any academics that were associated. This was really her idea. We did bring in a couple of co-founders to get their expertise and advice and help and so on. I mentioned Dr. Pam Silver early on, we did a collaboration with the Catholic University of Leuven, KU Leuven who had some beautiful data in this field of nuclear cytoplasmic transport. We worked with human Chuck on x-ray crystallography in making sure that our drug bound to the protein where we thought it didn’t, and it turned out that worked out. But we had to spend about a million dollars total before we got our first big investor. That consisted of about a half a million dollars of our money in cash, plus about half a million dollars in promises to a whole bunch of people. From lawyers to assay developers to chemists that were working all on the hope and promise that the shares that we intended to issue them someday would be worth something.
Michael: This is entrepreneurship. This is people who are getting up every morning and asking people to believe in them. And essentially working with blood and sweat equity to get into a company that frankly in biotech has, at the time we started the company, has one in a thousand chance of actually leading to a drug that ever gets approved. Those are the numbers, but it’s super cool when it works. And that’s why we have amazing new miraculous drugs so frequently these days. Is we have a lot of companies and we have a lot of people working on stuff, but most stuff doesn’t work.
Steve: I’m sorry. Did you say one in a thousand or one in a hundred? I couldn’t hear you.
Michael: From the time you come up with an idea and get started and put in capital, say more than a hundred grand, it’s about one in a thousand.
Steve: Wow, that’s incredible.
Michael: And by the time you even get into humans, just to bring it back to some of the numbers we talked about, if you’re in humans, you have about a one in 20 chance of being approved overall. One in two zero. So obviously your odds changed a lot. They went from 100,000 to one in 20, but they’re still not really great odds unless you have a strong stomach.
Steve: Now, in terms of this first million, in your case, is that a somewhat unusual story? What is a typical source for the first million or two on a company like this?
Michael: The stories are variable all over. We definitely were atypical because not only do we put … people put in their own money when they can. They get friends and family, sometimes they have what are called angel investors, and there’s a bunch of that kind of available thing around. But typically for a new idea … their also many new ideas come directly out of academic labs based on research and patent offices. And that can attract some academic funding and or NIH funding. And usually there has to be some proof of concept, either in a test tube or preferably an animal before you can get significant money to come in.
Michael: And when I say significant money, in biotech that’s typically a series A fundraising. So the series A will come after the “founders” or “angel investors.” And the foundering investments or the grant money will typically be on the order of a quarter million to a couple of million dollars. But if you want to progress beyond that, you really have to start to get into the typically 10 to even 70, $80 million investments.
Corey: And that’s where you typically raise a series A, Michael?
Michael: That’s right. So we raise a series A a little bit unusually. This whole project was unusual. Sharon, in true entrepreneurial and Israeli spirit I would say, did cold emails to about 10 of the best cancer researchers in Boston. And obviously we have lots of great educational institutions here, and she wrote to a lot of great people. One in particular, Dr. Ron DePinho, who was running one of the major institutes at Dana-Farber Cancer Center and went on to become the president of MD Anderson Cancer Center. Ron wrote back to her immediately and said, “This is a fantastic idea. I kind of wrote about this a few years ago, but I didn’t really come to the right conclusion. I love it, and I want to be involved big time.”
Michael: We met Ron and we were all very excited to work together. So Sharon and myself and Ron, and a couple of collaborators really got together. Several months after meeting Ron, he introduced us to one of his benefactors. One of the people that had donated money to the Dana-Farber that he knew, a multibillionaire named Wiaczeslaw Smolokowski, also known as Slava Smolokowski who was born in the Ukraine and lives primarily in Warsaw. We met Slava and he decided to invest $20 million in series A into the …
Michael: Slava, and he decided to invest $20 million in Series A into the company, and this was an amazing meeting. Basically, Slava said, “If Ron believes in the science, I believe in it.” But Slava got his very good friend, a guy named Dr. Minz or Raza Mirza to be in the diligence. And Mansoor is actually the Head of Gynecologic Oncology in the University of Copenhagen. So Mansoor did the diligence on the company, Mansoor remains a board member of Karyopharm, and he’s been a great advisor for us going forward. So, we really put together a nice group of people in a somewhat unusual thing.
Michael: Normally, you’ll get venture capital funding in the order of, like I said, about 10 to 80 million typical Series A, and the venture capitalists will come together, usually three or four of them, and they’ll join your board and you’ll have a very different kind of board than we did. We were lucky to have a single multi-billionaire investor along with his MD friend, and Ron DePinho, and his colleague, Julia [Driana 00:43:06], and myself, and Sharon.
Steve: So, at some point, will there be a visit to the public markets in your future? Or can you go the whole way without doing that?
Michael: No, it’s almost impossible. It took us about $15 million to get the drug into people, and it was lucky that the drugs started to show activity. We were a private company at that point, we had the one investment. We did a series B fundraising as the first glimpses that the drug might have activity and be tolerable occurred, and that was in 2012, late 2012. We raised about $42 million in a Series B fundraising, who was led by a friend of Slava’s. It’s one nice thing about wealthy people, they know other wealthy people who might be-
Steve: They all know each other.
Michael: They know each other. And this was [Grecia Yankelovich 00:00:44:04], and Grecia led that round. Slava came in, we had several other private individuals that came in. And we had our first venture capitalist come in to that round, it’s a woman I knew, [Deepa Paki 00:02:17] Nathan, who ran a partner at Delphi Ventures. Generally, venture capitalists are very, very bright people, they’re very driven, they’re very focused. It’s difficult though, because their focus is to make money and that’s appropriate, that’s their job, and that’s why people invest in their funds. Sometimes, that’s not commensurate with being able to do good drug development, it just makes it more difficult.
Michael: But I’ve worked with Deepa before she was involved in proteomics in Onyx, and she joined our board. And as the data started to come in, we had more and more interest, because when you start to have patients who have refractory cancers, meaning cancers whose disease had progressed despite of the best therapy we have now. And we were giving them oral… Our drug is taken by mouth, it has some annoying toxicities, but nothing very dramatic.
Corey: What are those toxicities? Before we go on?
Michael: Yeah. We are not trying to sugarcoat it. These are truly side effects as opposed to devastating toxicities, for instance, your hair does not fall out. We cause nausea, we cause people to lose their appetite, we cause fatigue, and we cause lower counts of primarily of their platelets, which are the pieces of cells in your bloodstream that help you clot. It turns out all of these side effects that we cause are reversible, and most of them are actually preventable. We have a slew of really good medicines to prevent nausea. So, once we were starting to see nausea in our patients, we said, “The doctors should strongly consider giving the patient anti-nausea agents, either one or two.” And for the patients who got the anti-nausea agents, their nausea went away.
Michael: For the fatigue, it’s a little tougher. A lot of the fatigue was turned out to be because their appetite was down. And we have, not only is it… You can have the conversation with folks, “Please try to eat, even when you’re not that hungry.” One thing is our drug… The anorexia, it goes away after a couple of months, but at the first two months, it can be really problematic. So we have drugs that stimulate appetite, and we gave those drugs and it prevented a lot of this, and then a lot of the fatigue went away. And for the patients who have low platelet counts, if you stop the drug, the platelet counts come back in two weeks and it’s fine. And luckily, most of the patients, nearly all, don’t have bleeding despite these low platelet counts, so that worked out.
Michael: In addition, there are some new drugs that can help you boost your platelet counts. So, all of the cases of our side effects are reversible, almost all of them are reversible and don’t really form toxicities, as opposed to some of the major toxicities you get with chemotherapy, which can be nerve damage, low white counts leading to severe infections, and this sort of thing. So, we were starting to see good activity, and we attracted some more investors in what became a series B prime, we call it. Sort of a B two, if you will. We raised about another 15 or 20 million that was unexpected. And one thing you learned in biotech is you raise money when you can, because if you try to wait till when you need it, you end up generally hurting the company.
Michael: And about a year after the series B, we were able to go public, take the company public. We had very nice clinical data in humans with refractory cancers, mostly in myeloma and lymphoma. And we did our IPO in 2013, and raised about $125 million there and have done several, what I call, secondary offerings since then, to the tune as I mentioned. We’ve raised today, I think as of most recently, we raised about $800 million. The drug was approved a year ago for a refractory myeloma and Corey mentioned that in the beginning. We had spent about $500 million from the time we started the company, until the actual approval and the first sale of Xpovio for patients with refractory myeloma.
Steve: Thanks. So thanks for going into that, and I don’t know if Corey, that was part of your plan, but I think a lot of listeners are just interested, especially if they come from more of the software or IT side of entrepreneurship, myself included, don’t really understand how drug discovery entrepreneurship works, and what the funding processes look like, et cetera, et cetera. So, just to make a comment on it, I think it’s amazing because you’re talking about a multi-year extremely complex, extremely capital intensive process to bring a drug to market. And it is really an incredible feat of modern market capitalism that it can do this, right? I mean, it can marshal half a billion dollars and get a 100 MD, PhD type people working for almost a decade. It’s just nice to step back and marvel at it.
Michael: Can I just add fuel to that fire? One of the most important things that modern capitalism and the US being absolutely the world’s leader, we’ve taken over from practically over other country now in new drug development. These are the miracles of modern day medicine. It’s astonishing what’s been able to be accomplished. These are extremely costly, they’re high risk, they’re crazy timelines like you said, but it is just a marvel that we can develop these compounds. We used to refer to the new antibiotics as Wonder Drugs.
Michael: And really some of the new cancer drugs, giving people years when it used to be six months death sentences, and grueling therapies have turned into chronic therapies for a lot of people, and I appreciate you saying that. And I do want to say that I’m very proud to be part of this industry and being able to support this, because I think we all need to realize that the things we’re all inevitably going to need as we grow older, are going to come out of these crazy funding, capital, labor, intellectual power, intensive areas.
Steve: Yeah. The software and other tech industry is very different because you know from the get-go, or very soon, whether the product actually could work. And it’s more a question of what is a market fit. Like, do people really want that app or that device or? So it’s a very different world. And less uncertainty and extending over, not usually not quite as long period of time. The question I wanted to ask is the following, so there’s always this issue of… The US spends so much of its GDP on healthcare, and there’s always this claim that, “Oh, we could just become a single payer system like the NHS in the UK, and we’d save everybody money.”
Steve: But then the question is, is a disproportionate amount of the downstream payoff for what investors are trying to get, say out of your activity, is that contingent on us having this very expensive healthcare system in the United States? If we went to single payer and cut the by factor to the fraction of GDP we were spending on healthcare, would it not slow down drug discovery and reduce drastically amount of capital available to people like you?
Michael: Yeah. I have a pretty strong opinion on this, and for people that are interested, there’s a book by a guy named Peter Kolchinsky, who is actually a trained PhD virologist, turned successful venture capitalist. But Peter’s book, Kolchinsky K-O-L on the last name, The Great American Drug Deal, makes really good point, and that is, number one, drugs are the only part of healthcare that whose cost eventually goes down, and the reason for this is our generic system and the fact that patents extend for 17 years from the time of the accepted filing, and nuanced, plus or minus a few years, depending on how long you spend in clinical trials. But the beauty of drugs is, is that they ultimately become generic.
Michael: And the most expensive total expenditure for our US drug system are all generic drugs, because they’re the antihypertensive, anti-diabetics, and so on, that are been off patent and are now made at pennies a pill. I think the most commonly prescribed drugs in the US are now the reflux medications that were branded prior to second, and now it’s pennies, you can get over the counter at CVS and so on. So, it is part of the drug deal that we have. If we want to continue innovation, and these are just facts, I mean people invest in things they think they can make money. Companies like mine get to be where they are, because we have a $1.3 billion market cap, we sold all of $35 million worth of Xpovio last year or to date, and eventually, it’ll become a lot more.
Michael: But we got to be at this market cap, because people expect that eventually we’re going to sell a lot of the drug. And that’s partly because the drug is priced at a high level, because it’s a cancer drug that can extend life, and it’s commensurate with the other drugs in its class. And frankly, it is partly to recoup the investments and support the next generation of drugs. So it is very simple, the reason the US is the leader in new drug discoveries, because we have the capital structure and the intellectual horsepower, but there’s a lot of places with intellectual horsepower that just don’t have the capital structure or the risk tolerance to invest in this field.
Steve: Well, I wanted to tease that out a little bit, because you could have the venture capital networks and the ability to invest in the human capital that will develop the drugs. That could, in principle, be different from the health system that is willing to pay a lot of money for a few extra years of life at the end of your life, after you’ve developed some kind of cancer, right? If you had a health system that just refuse to pay for that extra treatment, then none of this would work, right? Because, the return on investment for this just wouldn’t be sufficient to get the money in play, does that make sense? The question is, does there not have to be some huge economy that’s willing to, in the sense, overspend on last few years of life or some special cures in order to support this whole discovery infrastructure?
Michael: Yeah, there absolutely has to be, and we are it. We, in the US, support many institutions around the world. In fact, or de facto, or upfront about it, this is one that we support de facto. Europe has decided that they’re just not going to spend the money on this stuff. And if we, as small companies, had to rely on our European sales, dollar for dollar would be about one third of what we would get here and we would never get funded, we just wouldn’t. People would not focus on this stuff. People wouldn’t develop drugs for often diseases typically, because you’d have to charge a million dollars a patient to literally save their life. And, if you can’t get that reimbursed, it’s not a market that anybody would be willing to spend.
Michael: Like I said, we don’t have time to go into the whole economics of it here, but at the highest level, there’s no question that we, as the US, have made a decision that if we’re going to advance medicine, we have to be willing to pay these prices. And again, I stress that the one thing that public misses in all this, and all the discussions overlook, either passively or actively overlook, is the fact that drugs are the only component of healthcare system that you can actually reduce the prices. It’s highly unlikely that we’re going to start paying doctors half of what they make here now and continue to have the quality of doctors that we have.
Michael: In most other countries, they pay doctors a lot less than they pay in the US, except that in most of those other countries, the really good doctors become private doctors. And it’s like everything else in economics, there have to be incentives. The other last point on medicine, and even since I graduated at Hopkins in ’92, medicine has become amazing compared to where we were, and if we continue on the same rate, we’re going to be more amazing in 30 years and so on. But, medicine only gets better because we make incremental advances, and people pay for those. Used to be that heart failure, you died in a year. That way it used to be, now it’s five to 10 years. And the reason is, is because we made incremental advances with each new therapy coming, so now we treat heart failure with a cocktail of four or five different drugs, and all of them together have led to this five year survival.
Michael: HIV, as everyone knows, used to be pretty fatal within about a year. And AZT was a good first step, but it’s the more recent therapies over the last 10 years that have jumped on that and made it into a chronic disease where you can almost live a normal life for a normal lifespan, and I think we’re all very proud of that stuff. So we just should make sure we’re careful when we start to alter the economics, which allowed that to occur.
Steve: Yeah. I just wanted to emphasize that because I really think it’s under appreciated, right? I mean, now we have a description in your case, a very personal description of what it took to get this drug to market, how many years of effort, how many dollars of investor commitment, and then we can link it back to what normally seems like an outrageous thing. Whenever we quote the fraction of GDP that the US spends on healthcare, it seems like a scandal. If you just look at that number in isolation, it’s a scandal, right? Or if you say, “Hey, what fraction of dollars spent in our healthcare system or just spent at end of life to prolong some guy’s life for few years,” that also seems like a scandal, right?
Steve: But when you then combine it with your story, then you realize, “Oh, but yes, but after a while, when that drug goes off patent, it’s basically free for everybody else.” So, all future generations get those few extra years of life if they need it from that drug for pennies, right? And that whole story in its fullness, I think, is not understood by very many people.
Michael: Yeah. I think the goal is that in 10 years, we’re not doing things the same way. The goal is, just as we did with HIV, we started off with AZT. We had DDI, we had DTC and so on, and those have all been replaced by much better drugs. The question is, how long do you want to wait between the initial set of drugs and the second set of drugs that changed the game and now made HIV chronic? The same thing happened with hepatitis C virus, we had Interferon initially to treat it, tough stuff, worked some of the time, a lot of side effects. Then we added Reiber there and tough stuff, other side effects. Then we started to build other things and we started to get glimmers that we would get to longterm disease control, and now we have a cure for HCV, hepatitis C virus.
Michael: That stuff comes in increments. It isn’t like somebody woke up one day and said, “I’m going to cure hepatitis C virus.” It’s what we started off talking about. You don’t prove anything about diseases until you make drugs for them, and those drugs lead to new drugs, lead to new drugs. Same thing, I think in most of science, right? You stand on the shoulders of giants, and we say that all the time, but we forget in medicine that these incremental benefits lead to breakthroughs, we’ve seen that in cancer therapy as well. So, my hope is that medicine continues to accelerate its speed of change and the speed of improvement till it get to the point where we have diseases that are largely controllable for a long time, if not cured.
Michael: Eventually those drugs all become generic, so you’re not spending a lot of money as much anymore. And then, we have a lot of other things that we’ve got to deal with and you mentioned them. End of life care can be defined in different ways, certainly end of life care for COVID-19, just to take a topical example, we’re spending $4,000 or $5,000 a day for people to be on ventilators. I don’t think anybody in this country thinks that’s a bad use of $5,000 a day, even if anywhere between 20 and 50 percent of those patients are likely going to die.
Michael: But, I think that we’re spending a lot of money now in this crisis, just as we do for people with non COVID-19, end of life stuff, and these are difficult problems. There’s a difference between that acute problem of an acute virus in a respiratory failure and the end of life is… Our best ends estimates, and this is purely based on non-comparative studies, because that’s the only way to do them. We can probably, for some patients, we can add five or six months of life, where they would have been dead within months, now that’s going to be eight to 10 months or something. Is it worth the money you’re going to spend for those people? Most of the people taking our drug say it is, I would say pretty much everybody, because they can always decide not to. So we want to make those things available.
Corey: So Steve, I want to hop in here a little bit. Because Mike, if you step back in history a little bit, you’ll see that… And I used to get reaction to this, there’s a big push in the 90s by a lot of large drug companies to develop new drugs. And that seemed to fail in many, many cases, so the ecosystem changed. So in fact, what you’re describing of your history is really a product of, I think, late 90s, 2000, essentially having small companies take on risks for larger companies. Is that picture correct?
Michael: Yeah. I think that the emerging picture is, is that a lot of the entrepreneurship and risk taking gets done in small companies, not all of it. But the nature of new discovery, and I think, software engineers see this also, a lot of people do. It’s very hard to make breakthroughs by committing, right? You got to have people that are willing to say, “I’m going to figure out a way to make this work, or I’m going to go down with the ship trying.” And, that’s just not the way larger companies… I don’t believe in most any area work, typically. It’s a lot easier for a group of crazy people who have to risk everything to come up with a marvel idea for our drug, and this is the third company I’ve been in now, where we did crazy things and they worked.
Michael: One Corey mentioned didn’t work, so that was the fourth company, but three out of four ain’t bad. And everybody, when we started these projects, told us that we were crazy and that we were never going to be able to make the drugs. And if we did, they were going to be too toxic, and you just can’t do this and so you shouldn’t bother, and work on something else. And that’s what happens at larger companies. So you have to…
Michael: And that’s what happens at larger companies. So, you have to have this mentality that you’re going to figure out a way.
Steve: Yeah. You’re totally preaching to the converted on that topic. And in medicine, it’s easy to understand because, like you said, all kinds of things had to go your way for you to be the one in the 1,000 that succeeded. What’s more shocking is that, if it’s some kind of idea in computer science, or IT, or software, you would think the big company could figure it out and would go there and do it. But even that much smaller, more predictable jump in innovation is often absent in big organizations, and it takes a small startup to actually do it. That part is actually more counterintuitive to me.
Michael: Well, another example I’ll just tell you is that, we’re just less risk averse, and remember that almost every new innovation has 50,000 reasons why it isn’t going to work and about one… maybe one or two pathways to get it to work. Until you get it to work, and then everybody of course jumps on and says, “Great, of course, that’s great, it works.” So, not only is the initial discovery or idea easier in a small company, the kinds of risks we’ll take with regulators… And we are a heavily regulated industry. It’s very, very carefully… The oversight on our industry is extraordinary. And you can debate whether it’s too much or too little, but in general, if you believe, it’s about right. You’re talking about human health, and you don’t want to hurt people. On the other hand, you have to balance people who are destined to die with almost 100% certainty within a given period, and who want to try something new and their doctors agree. That’s an option we like to make available for people.
Michael: And so, a smaller company like ours will go and say to FDA or the European regulators and so on, “We want to try this on these patients, and we’ll make sure of this safety parameter, we’ll do this lab testing, and we’ll take the risk.” And there is always a chance the regulator’s going to come back and say, “No, we’re not going to let you do that. It’s too risky.” And if they don’t do that to you, then the institutional review board at the actual hospitals or clinics where you do the studies, or the ethics committees in Europe will come back and say that. Those… That kind of interaction in a large company, a large pharmaceutical company is often career-ending. So, if you do… If you suggest a risk and the risk turns out to be bad, that the risk wasn’t worth it, or the… You get a no, then your career is pretty much short at a large company, because you’re graded on moving things ahead and not causing problems.
Steve: Yeah. That’s exactly why even in software where things are more predictable, the big company often doesn’t innovate. It’s because the local incentive for the manager at the big company is quite different. And something that an entrepreneur is readily willing to take the risk on to move his little startup ahead just get stalled out in the big company.
Michael: Yeah.
Corey: So Michael, I’d like to… I want to shift into COVID because you’re actually in clinical trials with your drug in COVID, which is a really interesting twist. But before that, actually want to get… I want to get a sense of Sharon’s background, because she’s been an equal partner in this company. So how… What’s her background? What brought her to the point of being able to essentially come up with an idea for this drug, essentially at a kitchen table, and make it realistic?
Michael: Yeah. So, Sharon was… grew up in Israel, in a town called Ra’anana, which is right outside of Tel Aviv, and went to the Israeli army. So, she keeps me in line. She was a lieutenant when she graduated an officer. She graduated from the Israeli army, and then she went to Tel Aviv University where she got a PhD in Biophysical Chemistry, and an MBA as well.
Michael: And she was actually programming for Microsoft, specifically Microsoft Excel, when she had an offer to join one of the labs there for… to do a little bit more on PhD thesis, and work on the discovery of algorithms for predicting how to make new drugs quicker, the way we described at the beginning. So her training was in using modeling, using protein structure modeling, and binding energies with small molecules to predict, how do I get to a drug or a potential drug candidate way faster than the laborious and highly capital intensive efforts of what used to be robotics, where you’d isolate a protein, you would test… Maybe you could test 100,000 compounds at a time. She can test three million. The costs would be enormous in the tens of millions of dollars, including all of the startup fees and so on. And the problems with assays going wrong, and people getting sick, and all this stuff would be avoided. Now, you can’t completely avoid all that stuff, but reducing the problem from one of 100,000 or 200,000 starting compounds down to 50 compounds is enormous. And the cost goes down logarithmically as well.
Michael: So, that… Her background was there, and actually her PhD thesis at Tel Aviv led to this company called Predix Pharmaceuticals, where I became the CEO here in the U.S., and the research was being done in Israel. And she moved herself and her family over from Israel to the U.S. because she saw that research was going to stay in Israel, but development of new compounds for depression, anxiety, Alzheimer’s and schizophrenia were all going to be done here in the U.S.
Corey: Yeah. That’s where most of my familiarity is with these large companies working on neuroscience drugs, and just having large scale failure after large scale failure in the ’90s.
Corey: And so this company, Predix, they were started in Israel, and this… And-
Michael: Right, yeah.
Corey: And what the background of the company?
Michael: Well, it was… The idea was to come up with computer-based algorithms to discover drugs for a group of proteins called G protein-coupled receptors, GPCRs. GPCRs, no one talks about them in the normal every day, but people know about beta blockers, and many of the migraine drugs, drugs for nausea and so on, are all drugs that bind to proteins that fall into this class GPCRs. Until about eight years after her PhD thesis was done, no sort of normal GPCR structure had not ever been elucidated, no one had ever figured out what they actually looked like in three dimensional space.
Michael: And so, drug development was hampered, because if you do know the three dimensional structure of something, it’s like, if you can look inside of a lock, you can much more easily build a key. If you can’t see the inside of a lock, you basically create 100,000 keys and just try one at a time. And that was sort of the old school. And her idea to put it another way was to create a synthetic structure, a model of G protein-coupled receptors on the computer, to create these algorithms to figure out if this… the drug, which is the key, would fit into that lock, which is the synthetic structure, on the computer, and to use that as a way to jumpstart the whole drug discovery process for GPCRs.
Michael: And we were very successful with a very small company and minimal investment, less than $100 million. We were able to put four different novel drugs into the clinic. We had a fifth drug, which we sold to Amgen for $20 million. And we had a couple of other drugs, which we sold to GlaxoSmithKline, which are still being tested for schizophrenia and other diseases. But unfortunately, our first two drugs for anxiety and depression did not work. And the company, unfortunately, was unable to continue, because nobody wanted to invest.
Corey: Failed in the sense of, did not have efficacy, or too toxic or…
Michael: No, they were actually all… They all were… They were all not toxic. They had minimal side effects. They showed the right biological changes in terms of measuring chemicals in the bloodstream, but they just didn’t work on the diseases, on depression and anxiety, and a second one on Alzheimer’s. And it gets to that earlier discussion we had, that all of our models, of course, these drugs worked in, otherwise they would never have gotten into humans. But humans always saw us for a curve ball, and in these cases, the humans were smarter than our drugs, and all the biology work, and unfortunately none of the clinical medicine, panned out.
Corey: Got it. So, let’s turned to COVID. How did you come to start working on COVID? How did you first realize there might be a link between COVID and cancer that allowed a drug for cancer to work in a viral infection?
Michael: Yeah. When Sharon started the company, and we started to really read the literature, and actually one of the beauties of these kinds of compounds, even before they get into the clinic, we gave them out to every academic institution we could find that was interested in working on them, including in tests for antiviral activity, as well as anti inflammatory activity, things as far as lupus nephritis, other manifestations of lupus, models of arthritis, and models of influenza infection, respiratory syncytial virus and so on. So, we had this huge research effort going on in academic labs across the world, other models of neurodegenerative diseases, where we use those data to help partner our drugs to Biogen Corporation.
Michael: So, a lot of science was getting done, and really exploded the basic science understanding of this pathway across cancers, many different types of cancer, combinations of cancer therapies in viruses, in inflammatory diseases, and in neurological diseases. And this was all getting done. Now, we had a little trouble with the coronaviruses because, although we had tested them early, the SARS coronavirus-1, the one that was responsible for the corona outbreak in the year 2000 or 2001 in China, we were not able to show that we were killing the virus, because it turned out we were killing the cells that they were growing in. And the reason we were killing those cells that they were growing is because they were cancer cells. And we’re very good at killing cancer cells. So, we couldn’t distinguish whether we were killing the virus or the cancer.
Michael: And actually, it was Sharon again who had the sort of epiphany on a trip to Philadelphia about six weeks ago now, or eight weeks now ago, that she said, “I think we should have coronavirus activity, because a lot of these proteins that the coronavirus makes actually have to interact with XPO1, our drug target, in order to proliferate, in order for the virus to propagate.” And there were some publications that had just hit the literature, that said that XPO1 was an essential protein for viral replication. So, she sent our scientists back to collaborate and find cell lines that were not cancerous, where we could grow coronavirus. And Dr. Ralph Tripp at the University of Georgia, in his PL3 facility, his biological safety license level three, which is the Ebola virus level kind of thing, and the coronavirus level, actually showed for the first time that we had direct inhibitory activity, quite marked actually for coronavirus-2, SARS-COVID-2 replication. And that really got us started.
Michael: Now, the other part of it, sorry for the long winded answer, is that, a lot of the problems in coronavirus infections are the inflammation that the body does. And that’s true also in influenza. In fact, many people believe that the fundamental problem and the reason people die of influenza or corona, is because of the over exuberant, overstressed inflammation that our bodies carry out. And our drug was known to actually block some of those important pathways for inflammation. This was known for a long time.
Corey: Does that mean, in general, the anti-inflammatories are effective against these viruses?
Michael: And any… Well, the thinking is that, they are certainly used. There’s a big study going on with a drug called Actemra, which is an… Tocilizumab is the generic name. It’s an… It’s a drug that blocks the inflammatory protein IL-6, because IL-6 are very high in patients with coronavirus.
Michael: So, we also have other drugs that are being used, the TNF-alpha blockers, and other inflammatory protein, we have drugs that block those and so on. So, this was known. It turns out our drug blocks IL-6, it blocks TNF-alpha, it blocks IL-1, and it also directly blocks the virus. So, we had one of the unique situations with our drug, that even at low doses, it should actually both block inflammation and block viral propagation.
Corey: I want to stop you here for a second. The ILs, these are interleukins, right?
Michael: Yes. That’s correct.
Corey: Can you give us a quick explanation of what these proteins are?
Michael: Yeah. They’re… Interleukin comes from the idea of sort of intercommunication between the leukins, which are the white blood cells. Leuko means white. So these are proteins that are made by white blood cells, typically by lymphocytes or macrophages, and they sort of speak to each other, they’re communication proteins.
Michael: And the interleukins, for the most part, some of them are against inflammation, anti-inflammatory, but some of them are very pro-inflammatory. And many of them also cause severe fevers. And interleukin-6, for example, causes severe fevers. And this is the reason people with COVID-19 get high fevers, is because their interleukin-6 levels are high. But it’s never just one protein, because our immune systems are very complicated. So there’s a set of proteins, all of which have slightly different names, but are confusing. Interleukin-1 also has proinflammatory and high temperature, what’s called pyrogenic induction activity. And it’s important to block these at least to try to get symptoms down in patients with COVID-19.
Corey: So, you guys have been fast-tracked by NIH. And this is a pretty interesting story in of itself. Normally it takes quite a long time to get into clinical trial, but I understand that you guys are about to enter clinical trial for low doses of Xpovio.
Michael: Yeah.
Corey: So, how has the system changed, and how did you get your drug so far so fast?
Michael: Yeah. So, this is a lot of credit to the regulatory bodies, both in the U.S. and in Europe. And I have to say, FDA has been really remarkable in this regard. First of all, it’s an approved drug already. So, we knew at least in the setting of cancer, it was considered safe enough and effective enough to warrant treatment of humans. So, that makes it easier. But we generated a… the scientific basis for doing these studies. As I mentioned, largely from Sharon’s efforts, there was a number of publications that came out at about the same time, saying that XPO1 was an important target for this virus, as well as the actual data. And we have the anti inflammatory activity.
Michael: We put together a clinical protocol. We sent it down to the FDA, in this case, the Food and Drug Administration who regulates all-
Corey: So, what’s the clinical protocol?
Michael: It’s actually the instructions on how to use the drug and what kinds of patients to use it in.
Michael: So, we sent that down to FDA. It went to the division of virology, which is appropriate, because this is a viral infection, obviously. But given that the division of hematology actually knew about our drug and had approved it, the expertise at the FDA on our specific drug was in the division of hematology. So, the FDA did a very nice collaboration inside of itself, having the virologist work with the hematologists, and helped us to create a much better protocol.
Michael: The FDA probably knows more about this virus because they’ve got every company that’s working on it sending in information. Then, so the FDA knows more about it than most people. And they were able to apply a lot of their learnings to our protocol and come up with a really professional protocol, which is then… It’s about a hundred page document that is sent to doctors at clinical trial sites. These are typically academic hospitals. And the doctors read the protocols and they say, “I think this is very interesting. I want to use this here.” Of course, all these doctors are treating patients that have the SARS infection.
Michael: And within about four weeks, from the time we had an initial protocol to the time when we had our first patient actually dosed with low dose Xpovio, which is a record. These are typically six to nine month processes. And this is because people were working on weekends, and in some cases even overnight, to get these protocols reviewed, comments back to us. We, of course, worked almost 24 hours a day for many days in a row to get this stuff done because people were dying and still are.
Corey: So, what’s the state of the drug now?
Michael: So, the drug is currently in a phase II randomized trial. We don’t tell the doctors or the patients who’s getting placebo and who’s getting the active drug. And they’re getting a dose that’s about one third the dose of the approved cancer dose. This is a well tolerated dose. We know that now. We’ve enrolled about 80 patients, eight zero. We’re looking for about 250 patients total to be enrolled before we’ll know, with pretty good certainty, either that it helps or doesn’t help for this infection.
Corey: So, the… Can you tell us which patients is being used in?
Michael: Yeah. It’s being used in patients that have severe COVID-19, which is defined as people who need to be on supplemental oxygen. They have to be in the hospital. It’s initial use, and we’re continuing now, is patients who are not on invasive ventilation, which means people who do not yet have a breathing tube.
Michael: And unfortunately, we have to do it that way because it’s very difficult to know, in patients that are already on a breathing tube, if things have gone on for too long. And that’s just the unfortunate part of medicine, is that sometimes there are processes that are irreversible, and we just don’t know enough about this disease yet to know if it’s truly possible to reverse COVID-19 once someone’s on a ventilator.
Michael: The drug that just got approved on emergency use for approval or indication is Remdesivir, which is a pure antiviral drug. And it’s also been used in patients with earlier stage infection than what we’re treating. So, we’re allowing patients to be a bit sicker. Remdesivir showed a nice, although modest benefit, but it’s definitely a start, and it definitely gives people hope.
Corey: So I think I missed it. What’s the timeline for you getting results from this trial?
Michael: We’re hopeful that in the next couple of months we’ll have results. So, fairly quickly.
Corey: Got it. Steve, at last questions or not?
Steve: No, Michael, I really enjoyed our conversation, and I can turn my video back on now, because my kids were taking an AP test just while we were talking. So, I had my… I was trying to minimize my bandwidth, but it was great chatting with you and really wish you a well with your endeavors.
Michael: Pleasure. Really, it was a lot of fun guys. I really appreciate it.
Creators and Guests
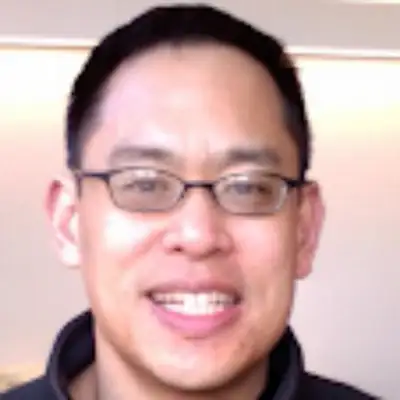